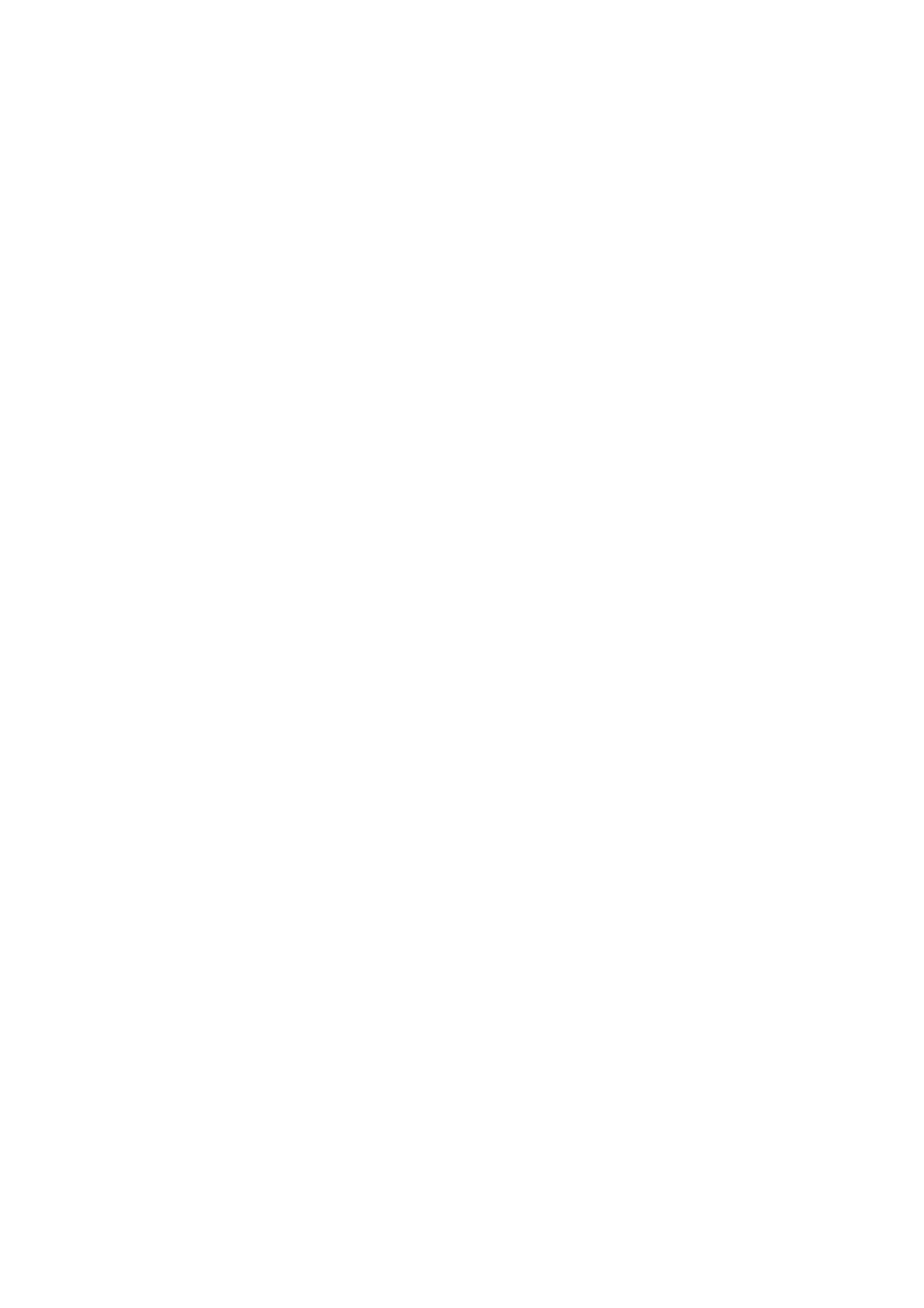
SUMMARY
This thesis describes the synthesis, characterisation and properties of phosphorus
containing ligands built on a calix[4]arene scaffold.
The first chapter describes the synthesis of bulky, monodentate phosphites. Reaction of
PCl3/NEt3 with p-tert-butyl-calix[4]-(OH)3-OR, in which R contains an auxillary O-donor (R = -
CH2P(O)Ph2, -CH2CO2Et, -CH2C(O)NEt2, -CH2CH2OMe) afforded the expected conical
calixarenes in which the P atom bridges three of the phenolic oxygen atoms. The thus formed
phosphites which have cone angles larger than 180° are remarkably stable towards aqueous
NaOH. When reacted with transition metal ions, they form either P-monodentate complexes or
large P,O-chelate complexes involving the oxygen atom of the R group. The four ligands were
assessed in the rhodium-catalysed hydroformylation of octene. Their activity is typically that of
a bulky phosphite, but the reaction rate decreases as the donor strength of the side group
increases. The L/B ratios lie in the range 1.4–3.6, the highest linear aldehyde selectivity being
observed when the side group is CH2CO2Et.
The second chapter deals with 6 calixarenes distally-substituted at the lower rim by two
PX2 groups (X = OPh, Ph), the other two oxygen atoms of the calixarene platform being
equipped with various substituents, R1 = propyl, R2 = CH2CO2Et, R3 = CO2cholesteryl. Reaction
of the di-P ligands with transition metals readily results in the formation of chelate complexes,
provided the reaction is carried out at high dilution. All ligands, when mixed with
[Rh(acac)CO)2], effectively catalyse the hydroformylation of octene and styrene. In the
hydroformylation of octene, the linear aldehyde selectivities observed with the phosphites
which contain R2 (L2) or R3 (L3) are significantly higher (linear/branched = ca. 10) than those
obtained with the other 4 ligands of this study and also with respect to PPh3. Molecular
modelling shows that the lower rim substituents of L2 and L3 form tighter pockets about the
metal centre than do the other ligands and so sterically favour the formation of Rh(n-alkyl)
intermediates over that of Rh(i-alkyl) ones. In styrene hydroformylation, all ligands result in the
formation of unusually high amounts of the linear aldehyde, the b/l ratios being close to 65:35.
The highest activities were found when using an L/Rh ratio of 1:1.
The final chapter describes the preparation of the diquinone obtained by chemical or
electrochemical oxidation of the proximally phosphorylated calixarene 1,2-(Ph2P(O)CH2)2-
calix[4]arene-(OH)2. As shown by an X-ray diffraction study, this calixarene adopts a partial-
cone conformation with one quinone ring being anti-oriented. In solution the two quinone rings
flip rapidly through the calixarene annulus.
Keywords: Calixarene, Transition metals, Phosphite, Phosphinite, Hydroformylation,
Calix-quinone