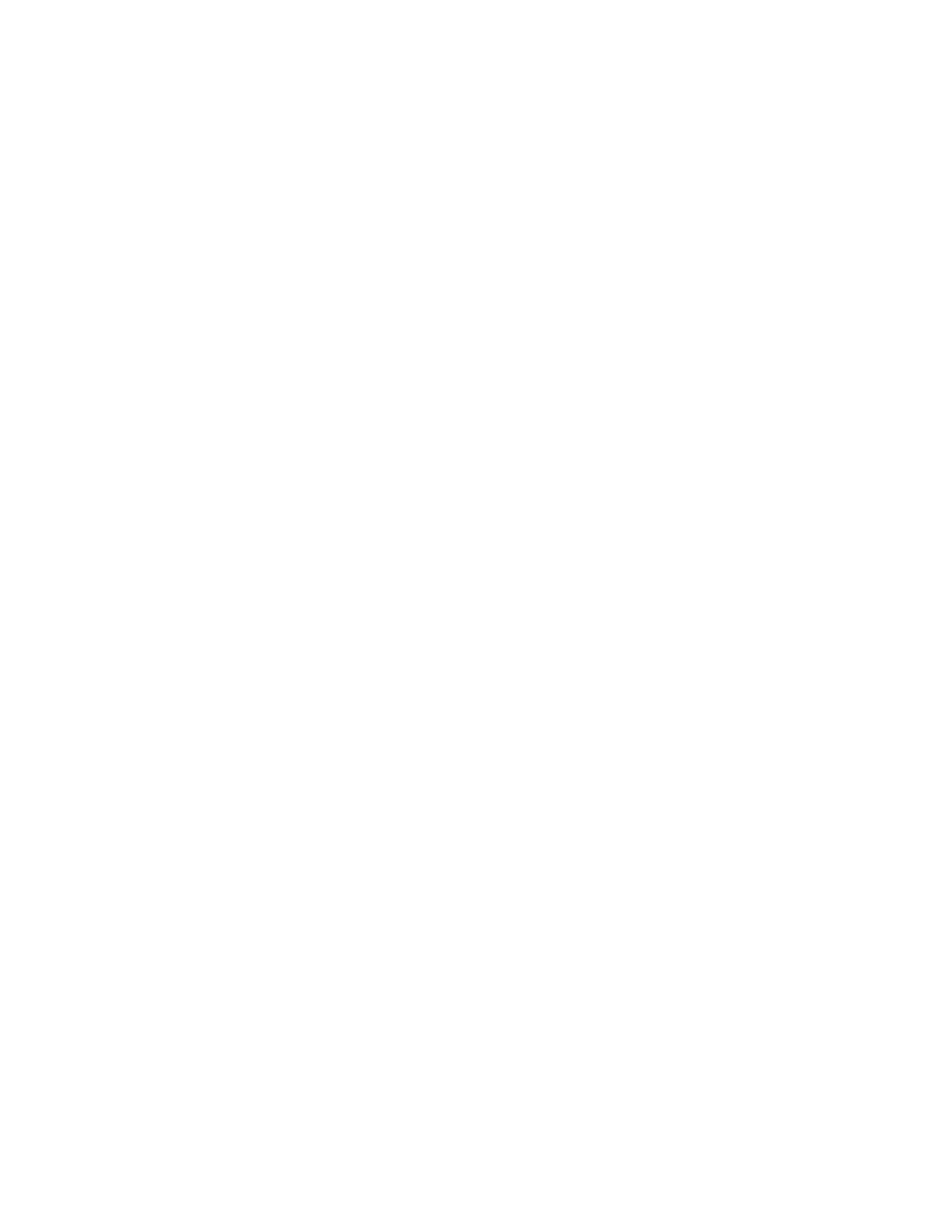
iii
Avant-propos
Après ces dernières années, je réalise à quel point la caractérisation de PNek1 s’est
avérée une tâche ardue. Je ne me suis jamais senti aussi près du but, mais aussi loin à la
fois. D’un point de vue forestier, j’ai débroussaillé pas mal large. Il reste par contre
beaucoup à faire. Je me réjouis toutefois que d’autres laboratoires aient commencé à
s’intéresser aux Neks. Ce projet m’a beaucoup apporté, tant du point de vue technique que
d’une meilleure connaissance de mes limites, mais surtout de mes intérêts. Bien que la
thèse soit l’élément final menant à l’obtention du Doctorat, je l’ai écrite pour moi. En ce
sens, cette thèse reflète bien mon esprit rationnel, ainsi que ma personnalité plutôt directe et
sans détour.
J’ai eu énormément de chance de travailler dans un environnement où l’accessibilité
aux ressources matérielles et surtout humaines n’était jamais une limite. Je te remercie
Armand de m’avoir donné cette chance et toute cette latitude de pouvoir orienter le projet
comme bon me semblait. Merci surtout d’avoir compris et supporté mes intérêts quant à
mon cheminement futur. Je ne crois pas que j’aurais pu me développer autant autrement.
Je tiens aussi à remercier John Mackay. Bien qu’on ne se croise
qu’occasionnellement, tu m’as prêté une oreille attentive. Tes conseils et ton expérience
furent très appréciés. Je suis bien content de te connaître.
Je remercie les membres mon jury de thèse, Dominique Michaud qui est là depuis le
début du projet, Michel Cusson que j’ai eu la chance de croiser au CFL, ainsi que François
Ouellet auquel je souhaite merde pour le début de sa carrière académique.
Merci aussi à Jacqueline Grima-Pettenati pour tes conseils et ta bonne humeur.
Louis Bernier, merci pour tes conseils avisés lorsque j’ai commencé ce projet.
Thank you Bob Rutledge for developing a technique which saved me several
months of work and for all those discussions; I learned a lot.
À l’équipe du labo, un gros merci. Caroline, Marie-Josée, Françoise, Gervais,
Dennis, Don et ceux qui ne sont plus là Laurence, Frédéric, Caroline. Merci pour tous les