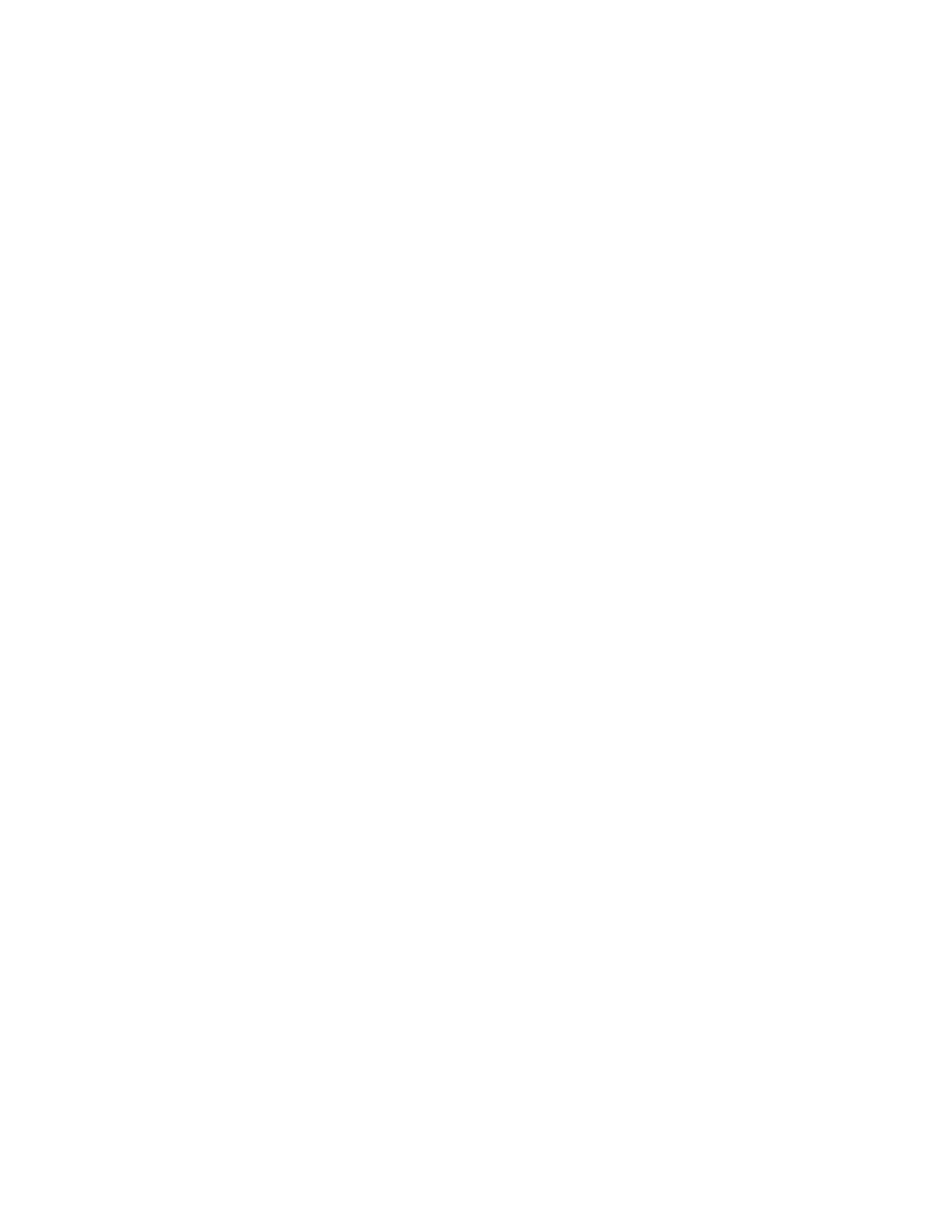
iii
Résumé
Les suppléments antibiotiques dans l'alimentation animale sont sévèrement critiqués. Les
probiotiques sont une alternative prometteuse, mais la caractérisation de leurs effets
demeure nécessaire. Ce mémoire décrit l'influence de Pediococcus acidilactici (PA) et
Saccharomyces cerevisiae ssp. boulardii (SCB) sur 1) le microbiote intestinal avant et
après le sevrage et, 2) l'immunité et la colonisation intestinale lors d'une infection à
Salmonella Typhimurium DT104. Nos résultats montrent que suite au sevrage PA module
le microbiote iléal de façon similaire aux antibiotiques tandis que SCB influence le
microbiote colique. De plus, SCB seul ou avec PA module certaines populations de cellules
immunitaires du sang avant et après l'infection à S. Typhimurium. Cependant, aucun effet
n'a été observé sur les autres paramètres évalués. Même si nous approfondissons la
compréhension entourant les effets de PA et SCB sur l'hôte, d'autres études sont nécessaires
pour optimiser l'utilisation des probiotiques comme alternative aux suppléments
d'antibiotiques dans l'élevage.