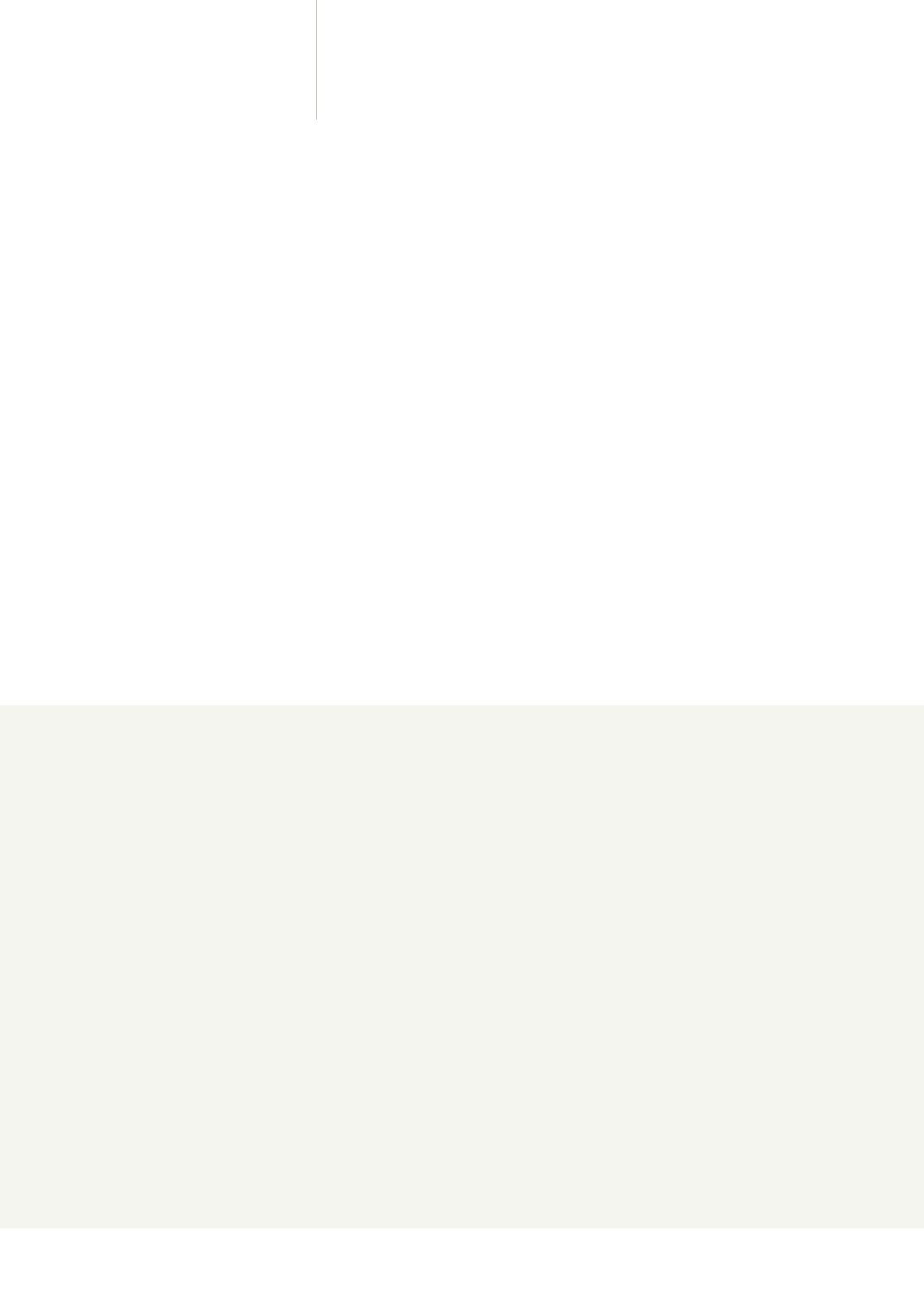
226 | La Lettre de l’Infectiologue • Tome XXVII - n° 6 - novembre-décembre 2012
DOSSIER THÉMATIQUE
Paludisme
Physiopathologie dupaludisme à
Plasmodium falciparum
:
principaux mécanismes et avancées récentes
Le ou les mécanismes par lesquels le jeune âge réduit
le risque de paludisme grave sont actuellement
inconnus (19). Il est possible que l’intensité variable
de cette rétention splénique détermine en partie
l’évolution ou bien vers l’anémie palustre grave du
nourrisson (rétention forte, évolution subaiguë, charge
parasitaire moyenne), ou bien vers le neuropaludisme
du jeune enfant (faible rétention, évolution rapide,
forte charge parasitaire) [29]. La rétention mécanique
est très probablement un déterminant important de
la circulation des gamétocytes et donc de la trans-
mission (28). L’expression de la famille multigénique
stevor est associée à ces modifications phénotypiques
(28, 31).
La rate joue aussi un rôle important dans la clairance
parasitaire sous traitement antipaludique, particu-
lièrement lorsque le traitement comporte un dérivé
de l’artémisinine (artésunate, dihydroartémisinine)
[32]. Lors du franchissement de la paroi sinusale
splénique, les corps figurés intraérythrocytaires non
déformables peuvent être expulsés du globule rouge
sans que ce dernier soit lysé. Ce processus original,
nommé épépinage (ou “pitting”), est mis en œuvre
physiologiquement pour éliminer du globule rouge
les résidus nucléaires. L’importance de ce phénomène
est abordée dans l'article consacré à l’utilisation de
l’artésunate i.v. en France.
Conclusion
La physiopathologie du paludisme implique donc
des mécanismes cellulaires, humoraux et immunolo-
giques qui s’avèrent complexes et intriqués. L’acteur
principal qui fait la spécificité de cette infection est
bien l’hématie parasitée qui interagit avec les cellules
de l’hôte. La rate est concernée par ces mécanismes
tout en exerçant un rôle de filtration et de régulation
cinétique. Tous ces mécanismes sont potentielle-
ment influencés par des polymorphismes de l’hôte et
du parasite, rappelant l’importance particulière de la
génétique dans l’expression de cette infection. Enfin,
ces mécanismes sont autant de pistes pour imaginer,
élaborer, voire évaluer de potentiels traitements
adjuvants (molécules diminuant la cytoadhérence,
immunomodulateurs, neuroprotecteurs, etc.). ■
1. Craig A, Scherf A. Molecules on the surface of the Plas-
modium falciparum infected erythrocyte and their role in
malaria pathogenesis and immune evasion. Mol Biochem
Parasitol 2001;115:129-43.
2. Crandall I, Sherman IW. Plasmodium falciparum (human
malaria)-induced modifications in human erythrocyte band
3 protein. Parasitology 1991;102:335-40.
3. Dondorp AM, Kager PA, Vreeken J, White NJ. Abnormal
blood flow and red blood cell deformability in severe
malaria. Parasitol Today 2000;16:228-32.
4. Dondorp AM, Desakorn V, Pongtavornpinyo W et al. Esti-
mation of the total parasite biomass in acute falciparum
malaria from plasma PfHRP2. PloS Med 2005;2:e204.
5. Schofield L, Hackett F. Signal transduction in host cells by
a glycosylphosphatidylinositol toxin of malaria parasites. J
Exp Med 1993;177:145-53.
6. Idro R, Jenkins NE, Newton CR. Pathogenesis, clinical
faetures and neurological outcome of cerebral malaria.
Lancet Neurol 2005;4:827-40.
7. Scuderi P, Sterling KE, Lam KS et al. Raised serum levels
of tumour necrosis factor in parasitic infections. Lancet
1986;2:1364-5.
8. Polder TW, Eling WM, Jerusalem CR, Wijers-Rouw M. A
cytochemical study of cerebrovascular lesions in mice infected
with Plasmodium berghei. J Neurol Sci 1991;101:24-34.
9. Orago AS, Facer CA. Cytotoxicity of human natural killer
(NK) cell subsets for Plasmodium falciparum erythrocytic
schizonts: stimulation by cytokines and inhibition by
neomycin. Clin Exp Immunol 1991;86:22-9.
10. Schofield L, Grau GE. Immunological processes in malaria
pathogenesis. Nat Rev Immunol 2005;5:722-35.
11. Barry OP, Pratico D, Savani RC, FitzGerald GA. Modula-
tion of monocyte-endothelial cell interactions by platelet
microparticles. J Clin Invest 1998;102:136-44.
12. Grau GE, Mackenzie CD, Carr RA. Platelet accumulation
in brain microvessels in fatal pediatric cerebral malaria. J
Infect Dis 2003;187:461-6.
13. Combes V, Taylor TE, Juhan-Vague I et al. Circulating
endothelial microparticles in Malawian children with
severe falciparum malaria complicated with coma. JAMA
2004;291:2542-4.
14. Faille D, Combes V, Mitchell AJ et al. Platelet micro-
particles: a new player in malaria parasite cytoadhe-
rence to human brain endothelium. FASEB J 2009;23:
3449-58.
15. Combes V, Coltel N, Alibert M et al. ABCA1 gene deletion
protects against cerebral malaria: potential pathogenic
role of microparticles in neuropathology. Am J Pathol
2005;166:295-302.
16. Pankoui Mfonkeu JB, Gouado I, Fotso Kuaté H. Elevated
cell-specific microparticles are a biological marker for
cerebral dysfunctions in human severe malaria. PLoS One
2010;5:e13415.
17. Snow RW, Marsh K. New insights into the epidemio-
logy of malaria relevant for disease control. Br Med Bull
1998;54:293-309.
18. Imbert P, Rapp C, Buffet PA. Pathological rupture of the
spleen in malaria: analysis of 55 cases (1958-2008). Travel
Med Infect Dis 2009;7:147-59.
19. Buffet PA, Safeukui I, Deplaine G et al. The pathogenesis
of Plasmodium falciparum malaria in humans: insights from
splenic physiology. Blood 2011;117:381-92.
20. Scherf A, Pouvelle B, Buffet PA, Gysin J. Molecular
mechanisms of Plasmodium falciparum placental adhesion.
Cell Microbiol 2001;3:125-31.
21. Groom AC, Schmidt EE, MacDonald IC. Microcircula-
tory pathways and blood flow in spleen: new insights from
washout kinetics, corrosion casts, and quantitative intra-
vital videomicroscopy. Scanning Microsc 1991;5:159-73;
discussion 173-4.
22. Ho M, White NJ, Looareesuwan S et al. Splenic Fc
receptor function in host defense and anemia in acute
Plasmodium falciparum malaria. J Infect Dis 1990;161:
555-61.
23. Looareesuwan S, Ho M, Wattanagoon Y et al. Dynamic
alteration in splenic function during acute falciparum
malaria. N Engl J Med 1987;317:675-9.
24. Safeukui I, Buffet PA, Deplaine G et al. Quantita-
tive assessment of sensing and sequestration of sphe-
rocytic erythrocytes by human spleen. Blood 2012;120:
424-30.
25. Buffet PA, Milon G, Brousse V et al. Ex vivo perfusion of
human spleens maintains clearing and processing functions.
Blood 2006;107:3745-52.
26. Deplaine G, Safeukui I, Jeddi F et al. The sensing of poorly
deformable red blood cells by the human spleen can be
mimicked in vitro. Blood 2011;117:e88-95.
27. Safeukui I, Correas JM, Brousse V et al. Retention of
Plasmodium falciparum ring-infected erythrocytes in the
slow, open microcirculation of the human spleen. Blood
2008;112:2520-8.
28. Tiburcio M, Niang M, Deplaine G et al. A switch in
infected erythrocyte deformability at the maturation and
blood circulation of Plasmodium falciparum transmission
stages. Blood 2012;119:e172-80.
29. Buffet PA, Safeukui I, Milon G, Mercereau-Puijalon
O, David PH. Retention of erythrocytes in the spleen: a
double-edged process in human malaria. Curr Opin Hematol
2009;16:157-64.
30. Keenihan SH, Gramzinksi R, Ratiwayanto S et al. Plas-
modium falciparum. Mechanisms of innate and acquired
protection against Plasmodium falciparum in Javanese
transmigrant adults and children newly resident in malaria-
endemic Northwest Papua. Adv Exp Med Biol 2003;531:
83-102.
31. Sanyal S, Egée S, Bouyer G et al. Plasmodium falciparum
stevor proteins impact erythrocyte mechanical properties.
Blood 2012;119:e1-8.
32. Chotivanich K, Udomsangpetch R, McGready R et al.
Central role of the spleen in malaria parasite clearance.
J Infect Dis 2002;185:1538-41.
Références bibliographiques