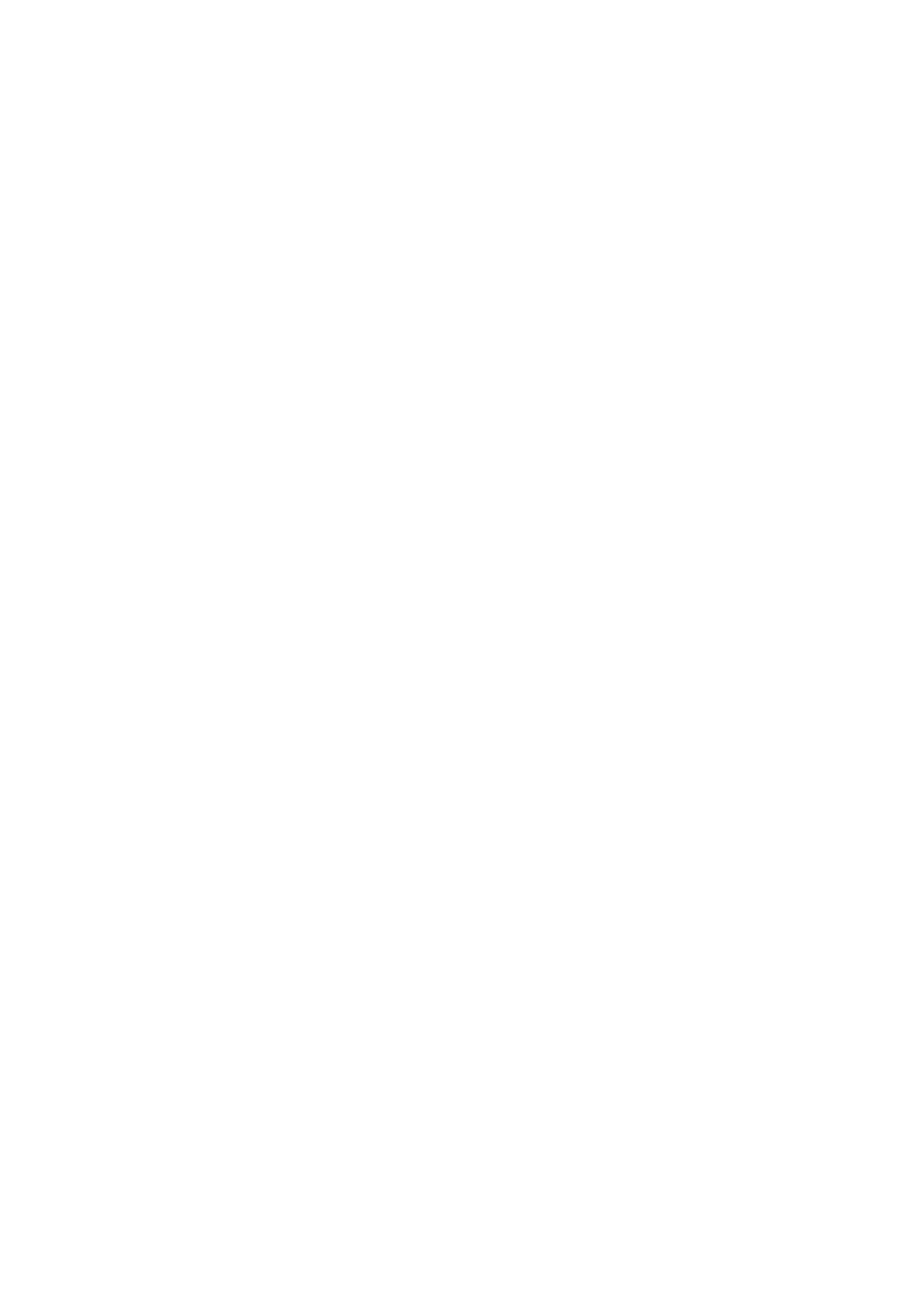
Table des matières
LISTE DES ABREVIATIONS..............................................................................................................7
LISTE DES FIGURES........................................................................................................................8
LISTE DES TABLEAUX.......................................................................................................................9
AVANT-PROPOS.................................................................................................................................10
CONTEXTE SCIENTIFIQUE …........................................................................................12
I. LES REPONSES T CD4 ANTITUMORALES ............................................................................. 13
1. ROLE DES DIFFERENTES SOUS-POPULATIONS DE LT CD4 DANS LES CANCERS ........................... 13
1.1. LES REPONSES T CD4 ACTIVATRICES ............................................................................................. 14
1.1.1. Différenciation et rôle des Th1 .................................................................................................... 14
1.1.2. Nouvelle population de LT CD4 à activité antitumorale : les Th9 ............................................. 20
1.2. LES LT CD4 ASSOCIES A UNE IMMUNOSUPPRESSION : LES TREG .................................................. 21
1.3. ROLE AMBIVALENT DES TH2 ET TH17 ............................................................................................ 28
1.3.1. Les lymphocytes Th2 .................................................................................................................. 28
1.3.2. Les lymphocytes Th17 ................................................................................................................ 32
1.4. AUTRES POPULATIONS DE LT CD4 HELPER ................................................................................... 37
2. HETEROGENICITE ET PLASTICITE DE LA POLARISATION DES LYMPHOCYTES T CD4 HELPER .. 38
II. LES ANTIGENES ASSOCIES AUX TUMEURS ...................................................................... 43
1. ROLE DES ANTIGENES TUMORAUX DANS L’IMMUNOSURVEILLANCE DES CANCERS ................... 43
2. CLASSIFICATION DES ANTIGENES TUMORAUX ............................................................................... 44
2.1. LES ANTIGENES MUTES A SPECIFICITE UNIQUE (TSA) ................................................................... 44
2. 2. LES ANTIGENES DU GROUPE CANCER / TESTIS ............................................................................... 45
2.3. LES ANTIGENES DE DIFFERENCIATION ............................................................................................ 45
2.4. LES ANTIGENES SUREXPRIMES ....................................................................................................... 46
2.5. LES ANTIGENES VIRAUX ................................................................................................................. 46
3. LA TELOMERASE: ANTIGENE TUMORAL CIBLE POUR LES LYMPHOCYTES T ............................... 49
3.1. STRUCTURE DE LA TELOMERASE .................................................................................................... 49
3.2. ROLE DE LA TELOMERASE DANS L’ONCOGENESE ........................................................................... 51
3.3. EXPRESSION ET REGULATION DE LA TELOMERASE DANS LES CANCERS ........................................ 53
III. PRESENTATION ET IDENTIFICATION DES PEPTIDES RESTREINTS PAR LE CMH
DE CLASSE II ..................................................................................................................................... 57
1. DISTRIBUTION ET REGULATION DE L’EXPRESSION DES CMH II .................................................. 57
2. LES VOIES DE PRESENTATION ANTIGENIQUE PAR LE CMH II ...................................................... 61
2.1. PRESENTATION DIRECTE DES ANTIGENES EXOGENES ..................................................................... 61
2.2. L’AUTOPHAGIE DANS LA CROSS-PRESENTATION DES ANTIGENES DE TUMEURS ............................ 65
3. STRATEGIE D’IDENTIFICATION D'EPITOPES T CD4 ANTITUMORAUX .......................................... 68
3.1. LA STRATEGIE PAR ELUTION DE PEPTIDES ...................................................................................... 69
3.2. APPROCHE BASEE SUR LES TILS ..................................................................................................... 69
3.3. CRIBLAGE DE LIBRAIRIES DE PEPTIDES CHEVAUCHANTS ............................................................... 69
3.4. STRATEGIE PREDICTIVE .................................................................................................................. 70