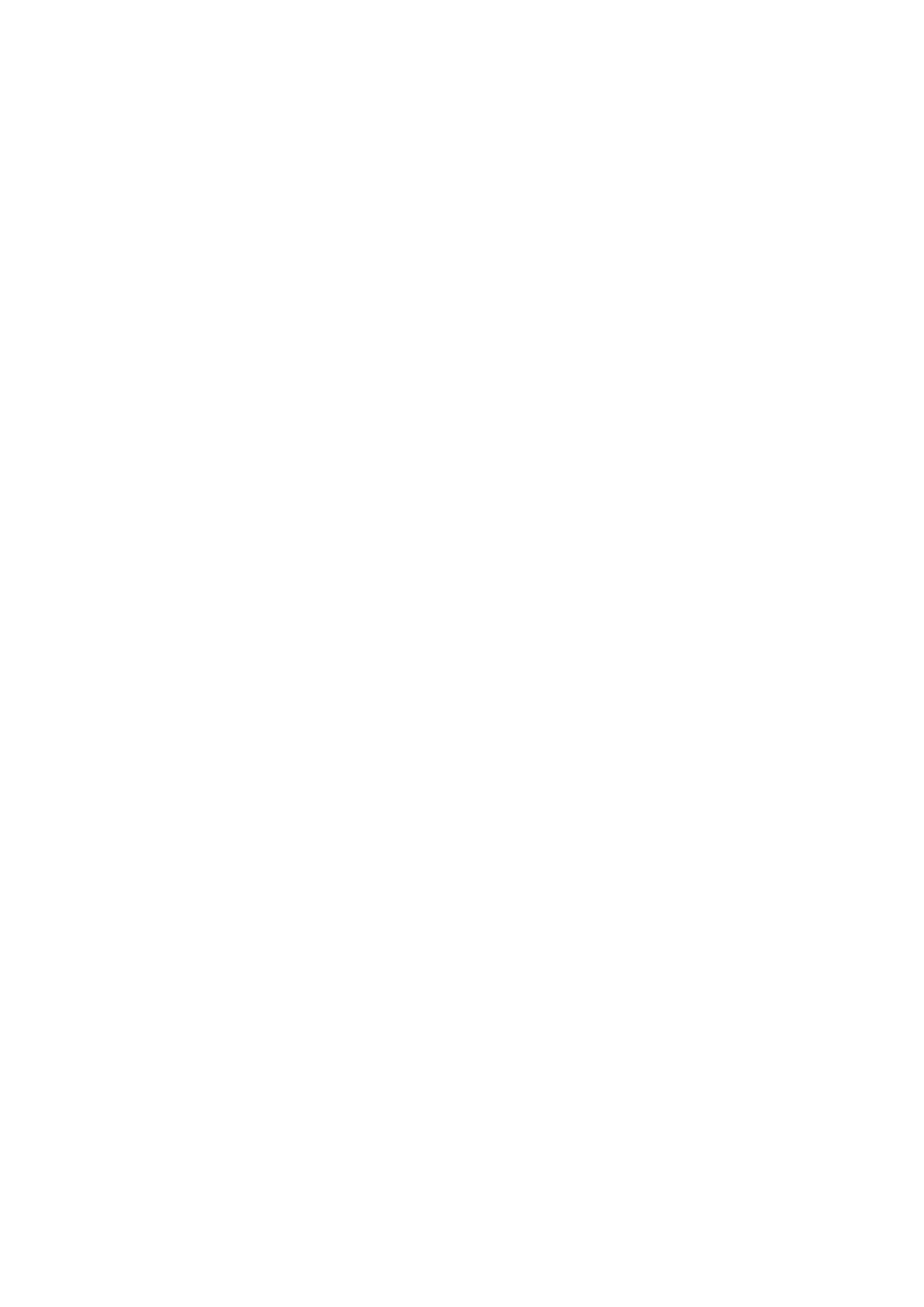
3
Contribution of niche modelling in conservation translocations: a case
study of reinforced populations of Houbara Bustard
Ecological niche models (ENM) are widely used to predict current and future
species distribution. By relating species occurrence with environmental variables,
these methods could be used as complementary tools to assess the ecological
suitability of potential release areas, a key parameter for improving success in
conservation translocation. Yet, ENM are still rarely applied in conservation
translocations and general evidence about the benefit of these models in translocation
is still lacking. The potential benefit of ENM is twofold by impacting both the
immediate success of translocations and the long-term population persistence in the
face of global change.
The aim of this thesis was to examine the theoretical and applied contribution of
niche modelling in species conservation translocation. We focused our research on
well-established reinforcement programs of two species of Houbara Bustard, the
sedentary North African species (Chlamydotis undulata undulata) and the migratory
Asian species (C. macqueenii). Through the extensive monitoring (by satellite and
radio-tracking) of remnant wild populations and captive-born released individuals over
a large proportion of their distribution range, these programs provide an ideal study
framework to address complex questions regarding the relevancy of ENM in
translocation conservation.
In a first part, we linked individual survival, a crucial component of population
dynamics, to habitat suitability as predicted from niche modelling. Despite the
increasing popularity of ENM for predicting current and future animal distribution,
few studies have provided empirical support on the relationship between habitat
suitability and bird survival.
In a second part, we investigated the contribution of niche modelling in
conservation translocations. We first performed an experimental release and
monitoring of 180 individuals along a gradient of habitat suitability as predicted by
ENM to test the effect of release site suitability on survival of captive-born
individuals. We then tested if released houbaras use the same ecological niche than
wild houbaras, as a validation step to support the subsequent use of niche modelling
within translocation programs.
In a last part, we used ENM to assess the potential impact of predicted climate and
land cover changes on Houbara distribution and dispersal. In light of our results, we
discussed the implications of global changes for ongoing translocation programs of the
sedentary and the migratory species of Houbara Bustard.
All together, this thesis clearly showed the contribution of integrating ecological
niche modelling techniques with conservation actions in reinforcement programs.
Empirical approaches linking habitat suitability with demographic processes (survival,
dispersal) or migratory routes provide a step towards a better understanding of the
relevance of ENM for conservation translocations in changing environments.
Keywords: ecological niche – global change – Houbara Bustard – niche
modelling – translocation