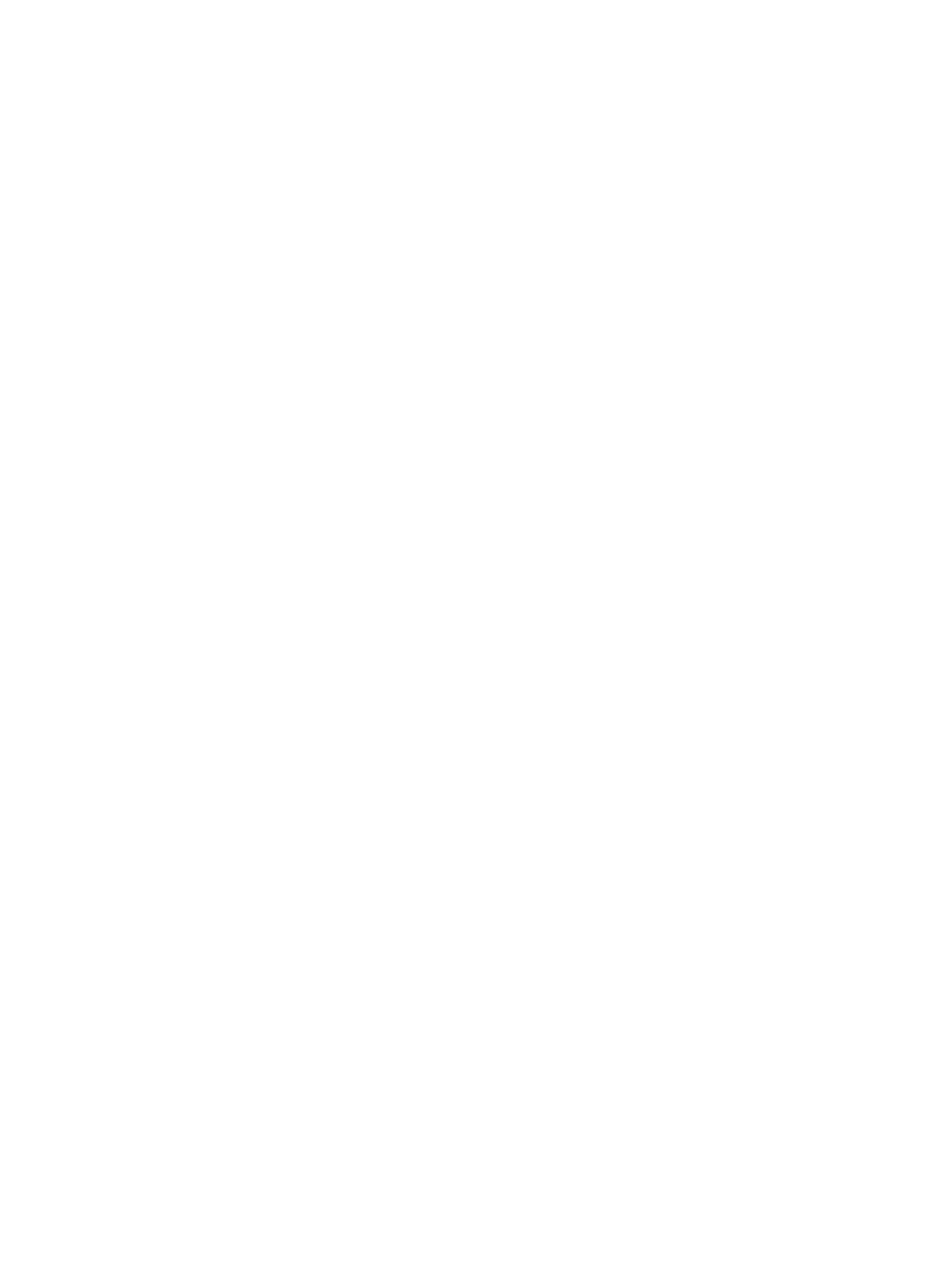
in S2, S3, or S4 were found to increase for some washing combina-
tions for all heavy metals of interest, indicating that portions of
these metals were redistributed during the washing procedure.
Some fractions were destabilized and readsorbed on the carbon-
ates, Fe/Mn/Al oxides, or SOM and sulfides. The residual metal frac-
tion (S5) was expected to be very stable and cannot generally be
removed or changed. However, in Exp. 7 of this study, where a high
PDTA dosage was employed, the S5 fraction of all four metals was
lower than it was in unwashed soil. Similar results were obtained
in a study of soil washing with EDTA (Lei et al., 2008).
A caveat that must be kept in mind when interpreting the re-
sults of the speciation experiments is that, as has been reported
in previous study (Yong and Mulligan, 2004), the determination
of heavy metal fractions by sequential extraction is only operation-
ally defined, and is generally considered to be more qualitative
than quantitative in providing insight into metal distribution and
mobility. The apparent metal redistribution revealed by sequential
extraction reflects only the increase in the chemical availability of
these metals after PDTA washing.
4. Conclusions
Heavy metal speciation and mobility in a contaminated soil
washed with PDTA, a derivative of EDTA, were investigated by
batch leaching tests using a range of soil washing conditions fol-
lowed by sequential extraction. With appropriate washing condi-
tions, PDTA significantly enhanced extraction of Cu from
contaminated soil. The primary mechanisms of Cu extraction by
PDTA were complexation-promoted dissolution of soil Cu and in-
creased dissolution of SOM. PDTA showed high selectivity for Cu(II)
over soil component cations (Ca(II), Mg(II), Fe(III), Mn(II), Al(III)),
especially at lower liquid-to-soil ratios under PDTA deficiency, thus
avoiding unwanted dissolution of soil minerals during the soil
washing process. PDTA-enhanced soil washing increased the
exchangeable fractions of Cu, Zn, and Pb and decreased their resid-
ual fractions, compared to their levels in unwashed soil.
A cost-effective strategy for the use of this agent would be a
continuous washing system where the contaminants were precip-
itated from the washing solution and the PDTA was reused. Soil
adsorption and biodegradation of PDTA should also be investigated
before this method is used in soil remediation.
Acknowledgements
The project was supported by National Natural Science Founda-
tion (No. 41171374, 21272292), National Funds for Distinguished
Young Scientists of China (No. 41225004), Guangdong Province
Higher Vocational Colleges & Schools Pearl River Scholar Funded
Scheme, the Ministry of Environmental Protection of China (No.
201109020) and the Research Fund Program of Guangdong Provin-
cial Key Laboratory of Environmental Pollution Control and Reme-
diation Technology (No. 2011K0007).
Appendix A. Supplementary material
Supplementary data associated with this article can be found, in
the online version, at http://dx.doi.org/10.1016/j.chemosphere.
2014.02.039.
References
Abumaizar, R.J., Smith, E.H., 1999. Heavy metal contaminants removal by soil
washing. J. Hazard. Mater. B 70, 71–86.
American Public Health Association (APHA), 1998. Standard Method for
Examination of Water and Wastewater, 20th ed. American Public Health
Association, Washington, DC.
Ball, D.F., 1964. Loss-on-ignition as an estimate of organic matter and organic
carbon in non-calcareous soils. J. Soil Sci. 15, 84–92.
European Chemical Bureau (ECB), 2004. European Union Risk Assessment Report on
Na
4
EDTA. Office for Official Publications of the European Communities, Italy.
Federal Remediation Technologies Roundtable (FRTR), 1994. Remediation
Technology Screening Matrix and Reference Guide, 2nd ed. DOD
Environmental Technology Transfer Committee, US.
Finzgar, N., Lestan, D., 2007. Multi-step leaching of Pb and Zn contaminated soils
with EDTA. Chemosphere 66, 824–832.
Khodadoust, A.P., Reddy, K.R., Maturi, K., 2004. Removal of nickel and phenanthrene
from kaoline soil using different extractants. Environ. Eng. Sci. 21, 691–704.
Kim, C., Lee, Y., Ong, S.K., 2003. Factors affecting EDTA extraction of lead from lead
contaminated soils. Chemosphere 51, 845–853.
Lei, M., Liao, B.H., Zeng, Q.R., Qin, P.F., Khan, S., 2008. Fraction distributions of lead,
cadmium, copper, and zinc in metal-contaminated soil before and after
extraction with disodium ethylenediaminetetraacetic acid. Soil Sci. Plant Anal.
39, 1963–1978.
Martinez, C.E., Motto, H.L., 2000. Solubility of lead, zinc and copper added to mineral
soils. Environ. Pollut. 107, 153–158.
Maturi, K., Reddy, K.R., 2008. Extractants for the removal of mixed contaminants
from soils. Soil Sediment Contam. 17, 586–608.
McBride, M.B., Sauve, S., Hendershot, W., 1997. Solubility control of Cu, Zn, Cd and
Pb in contaminated soils. Eur. J. Soil Sci. 48, 337–346.
Mulligan, C.N., Yong, R.N., Gibbs, B.F., 2001. Remediation technologies for metal-
contaminated soils and groundwater: an evaluation. Eng. Geol. 60, 193–207.
Nowack, B., Sigg, L., 1997. Dissolution of Fe (III) (hydr)oxides by metal-EDTA
complexes. Geochim. Cosmochim. Acta 61, 951–963.
Palma, L.D., 2009. Influence of indigenous and added iron on copper extraction from
soil. J. Hazard. Mater. 170, 96–102.
Palma, L.D., Ferrantelli, P., 2005. Copper leaching from a sandy soil: mechanism and
parameters affecting EDTA extraction. J. Hazard. Mater. B122, 85–90.
Palma, L.D., Mecozzi, R., 2007. Heavy metals mobilization from harbor
sediments using EDTA and citric acid as chelating agents. J. Hazard. Mater.
147. 768–755.
Peters, R.W., 1999. Chelant extraction of heavy metals from contaminated soils. J.
Hazard. Mater. 66, 151–210.
Pichtel, J., Vine, B., Kuula-Vaisanen, P., Niskanen, P., 2001. Lead extraction from soils
as affected by lead chemical and mineral forms. Environ. Eng. Sci. 18, 91–98.
Polettini, A., Pomi, R., Rolle, E., 2007. The effect of operating variables on chelant-
assisted remediation of contaminated dredged sediment. Chemosphere 66,
866–877.
Qiu, R.L., Zou, Z.L., Zhao, Z.H., Zhang, W.H., Zhang, T., Dong, H.Y., Wei, X.G., 2010.
Removal of trace and major metals by soil washing with Na
2
EDTA and oxalate. J.
Soil Sediment 10, 45–53.
Reddy, K.R., Chinthamreddy, S., 2000. Comparison of different extractants for
removing heavy metals from contaminated clayey soils. Soil Sediment Contam.
9, 449–462.
Sims, R.C., Sims, J.L., Sorensen, D.L., McLean, J., Mashmood, R., Dupont, R.R., 1984.
Review of In-Place Treatment Techniques for Contaminated Surface Soil-
volume 2: Background Information for In Situ Treatment. U.S. Environmental
Proteciton Agency, Washington, DC.
Tandy, S., Ammann, A., Schulin, R., Nowack, B., 2006. Biodegradation and speciation
of residual SS-ethylenediaminedisuccinic acid (EDDS) in soil solution left after
soil washing. Environ. Pollut. 142, 191–199.
Tejowulan, R.S., Hendershot, W.H., 1998. Removal of trace metals from
contaminated soils using EDTA incorporating resin trapping techniques.
Environ. Pollut. 103, 135–142.
Tessier, A., Campbell, P.G.C., Bisson, M., 1979. Sequential extraction procedure for
the speciation of particulate trace metals. Anal. Chem. 51, 844–851.
Theodoratos, P., Papassiopi, N., Georgoudis, T., Kontopoulos, A., 2000. Selective
removal of lead from calcareous polluted soils using the Ca-EDTA salt. Water Air
Soil Pollut. 122, 351–368.
Tipping, E., 2002. Cation Binding by Humic Substances. Cambridge University Press,
Cambridge.
Tsang, D.C.W., Zhang, W.H., Lo, I.M.C., 2007. Copper extraction effectiveness and soil
dissolution issues of EDTA-flushing of contaminated soils. Chemosphere 68,
234–243.
Tsang, D.C.W., Yip, T.C.M., Lo, I.M.C., 2009. Kinetic interactions of EDDS with soils. 2.
Metal-EDDS complexes in uncontaminated and metal-contaminated soils.
Environ. Sci. Technol. 43, 837–842.
Van Reeuwijk, L.P., 1992. Procedures for Soil Analysis, 3rd ed. International Soil
Reference and Information Centre, Wageningen, The Netherlands.
Wang, J.B., Qian, X.H., 2006. A series of polyamide receptor based PET fluorescent
sensor molecules: positively cooperative Hg
2+
ion binding with high sensitivity.
Org. Lett. 8, 3721–3724.
Yan, D.Y.S., Yip, T.C.M., Yui, M.M.T., Tsang, D.C.W., Lo, I.M.C., 2010. Influence of
EDDS-to-metal molar ratio, solution pH, and soil-to-solution ratio on metal
extraction under EDDS deficiency. J. Hazard. Mater. 178, 890–894.
Yang, Y., Ratte, D., Smets, B.F., Pignatello, J.J., Grasso, D., 2001. Mobilization of soil
organic matter by complexing agents and implications for polycyclic aromatic
hydrocarbon desorption. Chemosphere 43, 1013–1021.
Yip, T.C.M., Tsang, D.C.W., Ng, K.T.W., Lo, I.M.C., 2009. Kinetic interactions of EDDS
with soils. 1. Metal re-sorption and competition under EDDS deficiency.
Environ. Sci. Technol. 43, 831–836.
Yong, R.N., Mulligan, C.N., 2004. Natural Attenuation of Contaminants in Soils. Lewis
Publishers, Boca Raton, Florida, USA.
T. Zhang et al. / Chemosphere 109 (2014) 1–6 5