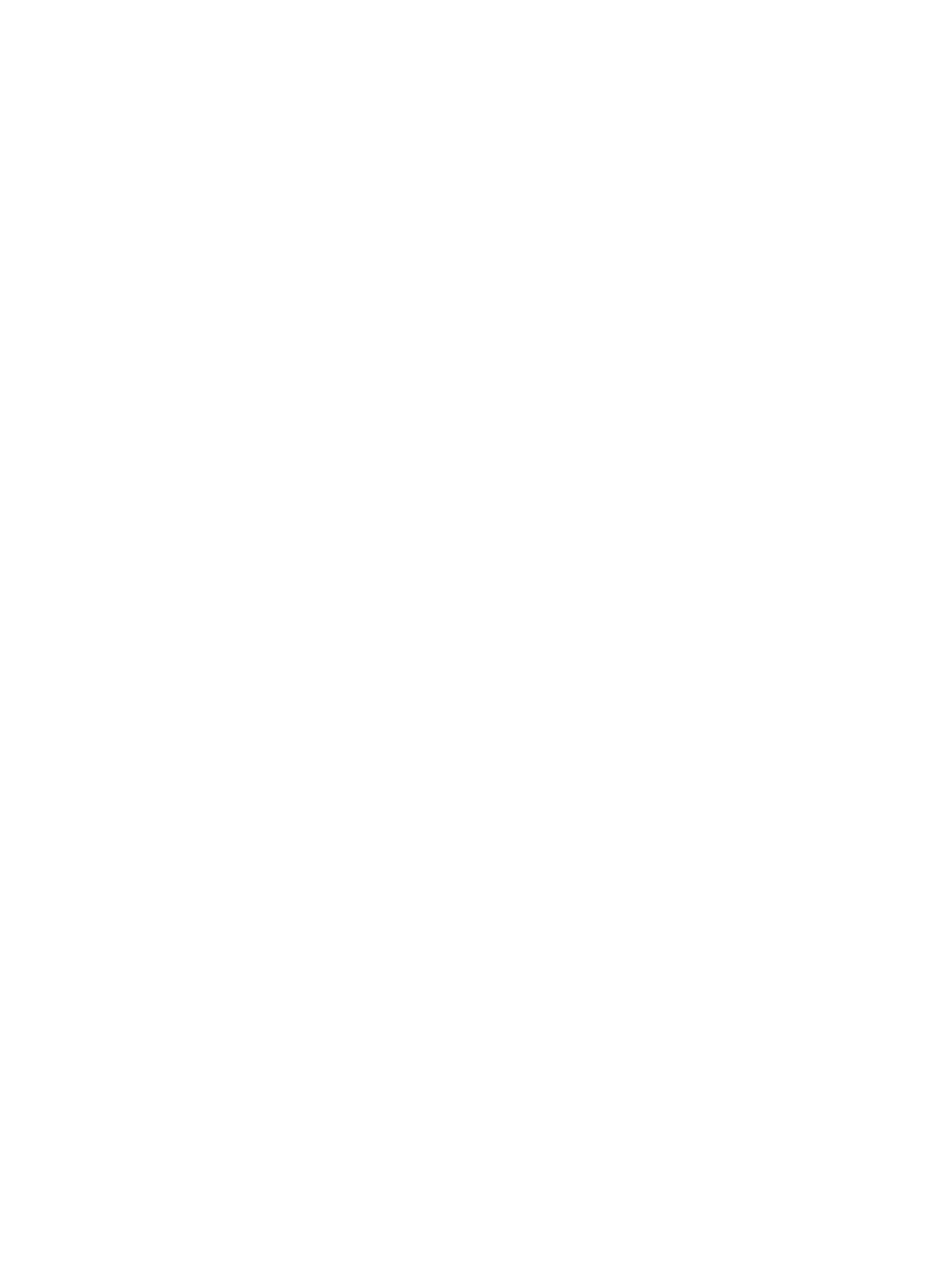
Mixtures covered in containers were steamed at 100
○
C for 20 min,
until starches in Mashed potato were fully gelatinized. After steamed,
gelatinized mashed potato was cooled to 26
○
C to prepare for printing.
Both of the screw-based and syringe-based printers were controlled
by a computer installed with Repetier Host V2.0.5 and Slic3r software
(Hot-World GmbH & Co. KG, Willich, Germany). The printed model was
downloaded from internet as a stereolithography file (.stl), then loaded
into the Repetier Host software and sliced by the Slic3r software into G-
code. The printing head was controlled in X, Y and Z axis by the G-code.
A 1.0 mm diameter nozzle was used in this study for both screw-
based and syringe-based printers. The nozzle height, extrusion rate, and
nozzle movement speed for all printing experiments were all set at same
values according to pre-tests.
2.2.3. Rheological properties measurements
The rheological property of mashed potato was carried out by using
a hybrid rheometer (Discovery HR-3, DHR, TA Instruments, USA) in-
stalled with a parallel plate with diameter of 20 mm and a gap of
1000 μm at 25
○
C. Mashed potato samples were loaded in the gap and
were allowed to rest for 10 min before measurement to achieve a stable
measurement results. The storage modulus (G′) and viscous modulus
(G″) measurements were carried out by a dynamic oscillation frequency
analysis with 0.1% strain and with linear viscoelastic range with fre-
quency of 0.01–16 Hz. A sweep flow analysis was conducted at a shear
rate range from 0.001 to 10 1/s for obtaining viscosity property.
2.3. Data analysis
Data analysis was carried out with the SPSS 20.0 software (IBM,
Chicago, IL, USA). All experiments were performed in triplicates.
3. Results and discussion
3.1. Simulated velocity profile
Velocity profile of syringe-based 3D food printer was simple top-
down laminar flow (Fig. 3a). The velocity of fluid in the cylinder was
relatively slow but uniformly distributed. Starting from the bottom part
of the syringe, the flow line concentrated towards the center and its
velocity gradually increased from the top to the bottom of the nozzle,
with a maximum velocity at the outlet of nozzle. This simple, top-down
flow field may cause an accumulation of dispersed phase in the multi-
phase ink at the junction of the cylinder and the nozzle. As shown in
Fig. 3b, the velocity profile of screw-based 3D food printer was com-
plex. High velocities were found around the screw, while fluids in
nozzle showed low velocities. Most of the fluid in extrusion tube pre-
sented rotating around the screw with higher velocities. Note that, a
vortex occurred at the top of nozzle, which can stir inks for a better
uniformity. These velocity profiles appeared in extrusion tube and
nozzle show a potential for the printing of non-homogenous (multi-
phases) inks, for example the mixture of solids and liquids.
As shown in Fig. 3c, the longitudinal velocity field distribution in
syringe-based 3D food printer was homogenous at the cylinder and
increased from top to bottom of the nozzle. Moreover, the velocity of
fluid in the nozzle gradually rose from the boundary to the center. This
signified that the velocity of inks at the exit distributed non-uniformity,
which may cause a jet expansion affecting the printing. On the contrast,
the longitudinal velocity field distribution in screw-based 3D food
printer was more complex than that in syringe-based printer (Fig. 3d).
High longitudinal velocities were found under the screw thread, while
some backflow occurred at the gap between walls and the screw flights.
For powder-feeding printing, for example chocolate printing, once the
molten materials flows back to the lower temperature region, it will
solidify and affect the overall printing process (Tseng et al., 2018).
Thus, reducing the gap between walls and the screw flight in the ex-
trusion tube can be an efficient way to improve the capacity of the
screw-based 3D food printer for high viscosity inks. Another way to
improve the extrusion performance is to reduce the viscosity of inks.
Whereas, this measure may result in a reduction in the mechanical
strength of inks, thereby reducing the precision of the extruded object
and its supporting capacity (Liu et al., 2018b). The syringe-based 3D
food printer is a better way to overcome the back flow issue in the
printing process of high viscosity inks (Vaezi and Yang, 2015). Liu et al.
(2017) also suggested that the syringe-based and air pressure-based
extrusion units are more suitable for the materials with high viscosity
and mechanical strength than screw-based extrusion unit. In another
note, the syringe-based and air pressure-based 3D food printer have
their natural drawbacks, for example the batch printing (Liu et al.,
2017). Screw printing can operate continuously as it enables to feed the
ink material through the inlet hopper.
3.2. Simulated shear rate distribution
The rheological and viscosity properties of inks are critical for a
precision and accuracy in printing (Godoi et al., 2016). Among these
parameters, the viscosity directly affects the ink extrusion effect. A
desirable low viscosity is crucial for providing a continuous extrusion
(Liu et al., 2017). Whereas, some edible inks, such as mashed potato,
fish surimi gel and lemon juice gel, are pseudoplastic or shear-thinning
fluids (Liu et al., 2018b; Wang et al., 2018; Yang et al., 2018a). The
shear rate distribution in extrusion tube/syringe is crucial for under-
standing the state of fluid, for example the viscosity, thus for better
control the extrusion process.
As shown in Fig. 4a, the shear rate of fluids in the syringe cartridge
distributed homogenously with a low value. High shear rate values
were found around the outlet of nozzle, rapidly increasing from top to
bottom. These high shear rates could decrease the viscosity of shear-
thinning inks to a relatively low value. Thus, the ink could be easily
ejected through the tiny nozzle outlet (Shastry et al., 2006). As for the
screw-based 3D food printer, most of the shear rate values were
homogenously distributed in the extrusion tube. Whereas, some high
shear rate values were found at the gap between walls and the screw
flight (Fig. 4b). This simulated result is similar with the previous si-
mulation study on screw extrusion (Singh Sushil and
Muthukumarappan, 2016). During extrusion, the screw spins rapidly to
provide a push for ink. As a consequent, inks at the gap will suffer a
high shear rate by the spinning flight. These high shear rates thin the
inks at the gap resulting in an idle turning of the screw.
3.3. Simulated pressure distribution
As shown in Fig. 5a, the pressure distributed homogenously in the
cylinder of syringe-based 3D food printer, while decreased rapidly near
the outlet of nozzle. The pressure gradient between the nozzle and the
cylinder ensured that the ink can be extruded smoothly. Inks in cylinder
suffered the highest pressure among the printer, which is directly ap-
plied through the plunger of syringe. For printing the inks with high
viscosity, higher power needs to be applied to the motor of printer (Sun
et al., 2018). For screw-based 3D food printer, the pressure distributed
homogenously in the nozzle. However, some negative pressure values
were found above the screw flight at the top of the extrusion tube
Fig. 5b. This negative pressure forms a pressure gradient with atmo-
spheric pressure, allowing the ink to be fed continuously from the
hopper. However, the pressure of fluids in extrusion tube increased
from top and was found highest at the bottom of the extrusion tube and
nozzle. This simulated result indicated that a backflow may happen
when the pressure at the nozzle point is too high which corresponds
with the results of simulated velocity mentioned above. From the
C.-F. Guo, et al. Computers and Electronics in Agriculture 162 (2019) 397–404
400