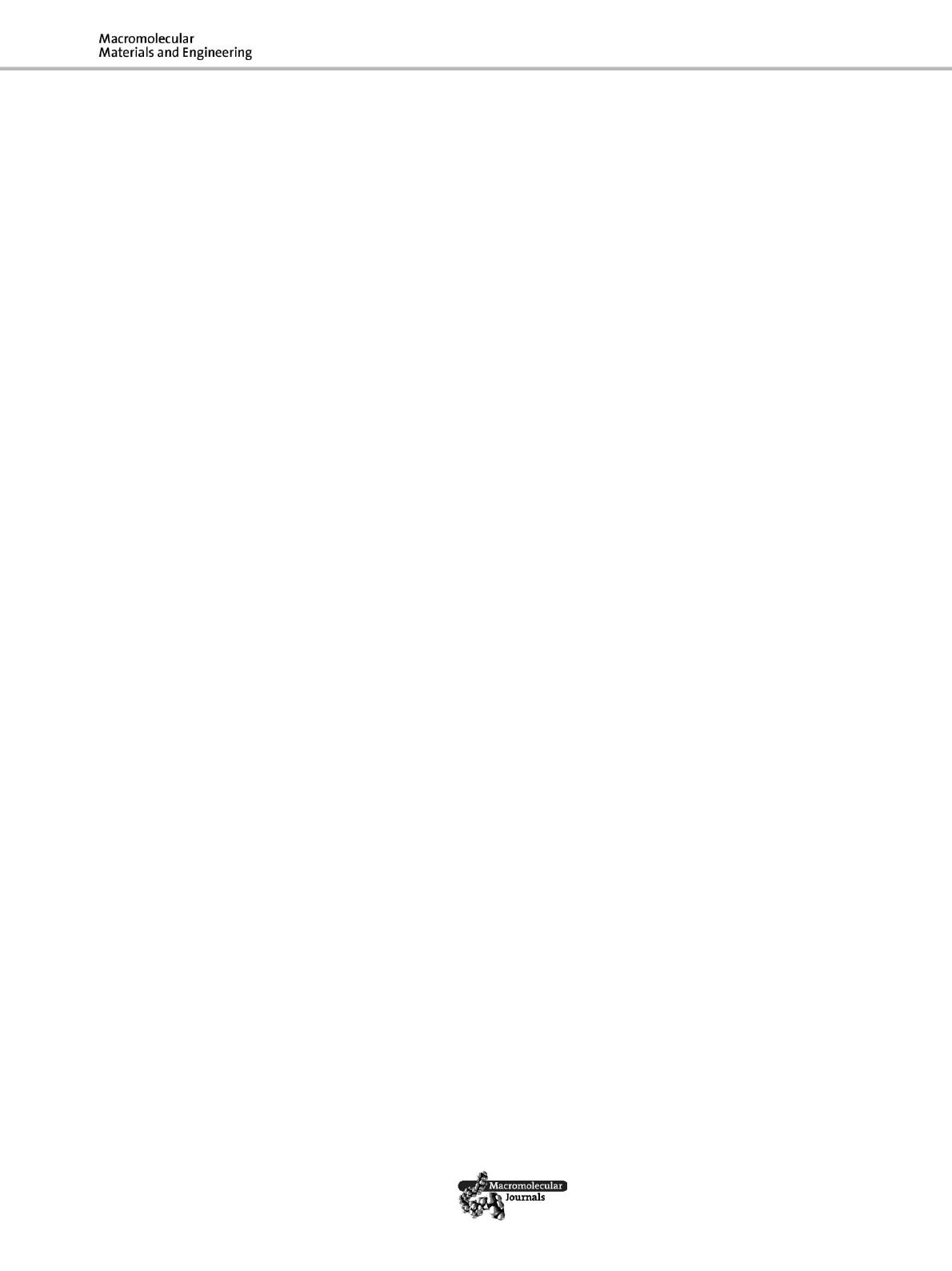
agent, such as sodium sulfite, these cross-links can be
broken, greatly improving processability. It was shown that
the apparent viscosity during extrusion, decreased with
increasing sodium sulfite up to 3 wt.-%, after which it
increased with the addition of more sodium sulfite. It was
hypothesized that the increase in viscosity after 3 wt.-%
was due to increased chain mobility and entanglements
after the complete reduction of disulfide bonds. It was
found that protein chain mobility was sufficient
above 4 wt.-% sodium sulfite, to allow chains to be
orientated into crystalline structures, as revealed by NMR
spectroscopy.
[22]
Orliac et al., investigated the rheological behavior of
sunflower protein isolate (SFPI) processed with water,
glycerol, and in some cases sodium sulfite. It was shown
that sodium sulfite can be used to reduce the required
amount of plasticizer for thermoplastic extrusion. An initial
decrease in viscosity was observed, followed by an increase
upon further addition of sodium sulfite. It was concluded
that greater protein unfolding at higher sodium sulfite
concentrations, led to a more extended structure after
processing.
[30]
Ralston and Osswald
[31]
used a screw-driven capillary
rheometer to measure the viscosities of soy protein isolates
extruded with cornstarch, glycerol, sodium sulfite, de-
ionized water, and soy oil. In the absence of sodium sulfite,
disulfide bonds reduced the effective chain length and ledto
a more globular protein conformation.
[31]
Soy protein does
not have extensive cross-links, and processing is therefore
not hugely influenced by the addition of sodium sulfite.
However, it was found that the use of sodium sulfite
increased consolidation by enabling chain movement. This
will lead to exposure of functional groups capable of
forming new interactions.
The viscosity of a polymer melt depends on protein
composition. Covalent cross-links are not always the
inhibiting factor. Water insoluble and hydrophobic pro-
teins, such as zein, require the addition of surfactants to
enable thermoplastic processing. Sodium dodecyl sulfate
(SDS) is an amphiphilic molecule, capable of electrostatic
and hydrophobic interactions, causing dissociation of
protein chains. Many studies of protein-based plastics
formed by cast and compression molding have used SDS for
added denaturation and dissociation, thereby improving
processing.
[32–38]
Sessa et al.
[38]
studied the viscosity of zein during torque
rheometry. Known amounts of water, triethylene glycol
(TEG), and SDS were added in order to control viscosity. The
addition of water resulted in a rapid torque rise after 1 min.
Further addition of TEG and SDS delayed the rise by up to
12 min. They postulated that at a certain temperature and
shear, zein denatures and forms entanglements and
aggregates leading to a rapid increase in torque. It was
concluded that the reduction in intermolecular forces,
brought about by the presence of SDS, slowed the rate of
aggregation induced by processing.
[38]
Urea can be used to denature a protein molecule, and is
extensively used in proteomics.
[39]
It forms hydrogen bonds
with amino acids, thereby preventing protein-protein
interactions. The majority of bioplastic research using
urea, has used compression molding as a processing
technique. Mo and Sun
[40]
investigated the thermal and
mechanical properties of plastics molded from urea-
modified soy protein isolates. Urea increased the degree
of denaturation of soy protein and the glass transition
temperature (T
g
) decreased with increasing urea concen-
tration, but mechanical properties reached a maximum
value at 8 mol L
1
. At low concentration (X<2 mol L
1
),
urea functioned as a plasticizer, while at high concentra-
tions (X>4 mol L
1
) it acted as plasticizer, cross-linking
agent, and filler.
At high concentrations, urea can form a surface residue
after long-term storage. Pommet et al.
[19]
used 30 wt.-% urea
in wheat gluten and Mo and Sun
[41]
investigated the effect
of storage time on urea-modified soy protein, both
observing the formation of urea on the surface of the
material overtime. This shows that protein-protein inter-
actions are stronger than urea-protein interactions, forcing
the urea out of the material overtime.
[41]
Compared to
polyol plasticizers, urea is not as flexible and the plasticized
materials do not show the same extensibility. Therefore
urea is good as a denaturant, to increase chain mobility
during processing. The use of a stronger plasticizer may
further reduce strong protein-protein interactions which
could enhance urea diffusion to the surface.
Visco-Elasticity
Denaturation and therefore chain mobility, is essential for
successful extrusion. Proteins are semi-crystalline poly-
mers and undergo several thermal transitions, similar to
synthetic polymers. The most common techniques used to
measure the glass transition temperature of polymers
include differential scanning calorimetry (DSC) and
dynamic mechanical thermal analysis (DMTA). The glass
transition appears as a step in the DSC baseline, resulting
from a change in heat capacity (enthalpy). DMTA measures
the change in viscoelastic properties of the polymer with
changing temperature.
[43]
The glass transition temperature
is interpreted from the storage and loss moduli data
obtained from a DMTA run.
Thermal transitions are related to chain mobility, most
important of which is the glass transition (T
g
) which is
associated with the onset of main chain motions
(Figure 1).
[9]
It is the primary structure of a protein that
determines its tendency toward cross-linking, as well as its
sensitivity toward plasticization, or chain mobility.
[33,72]
Secondary transitions (short range motions) have also
C. J. R. Verbeek, L. E. van den Berg
14
Macromol. Mater. Eng. 2010,295, 10–21
ß2010 WILEY-VCH Verlag GmbH & Co. KGaA, Weinheim DOI: 10.1002/mame.200900167
14392054, 2010, 1, Downloaded from https://onlinelibrary.wiley.com/doi/10.1002/mame.200900167 by EVIDENCE AID - BELGIUM, Wiley Online Library on [05/12/2022]. See the Terms and Conditions (https://onlinelibrary.wiley.com/terms-and-conditions) on Wiley Online Library for rules of use; OA articles are governed by the applicable Creative Commons License