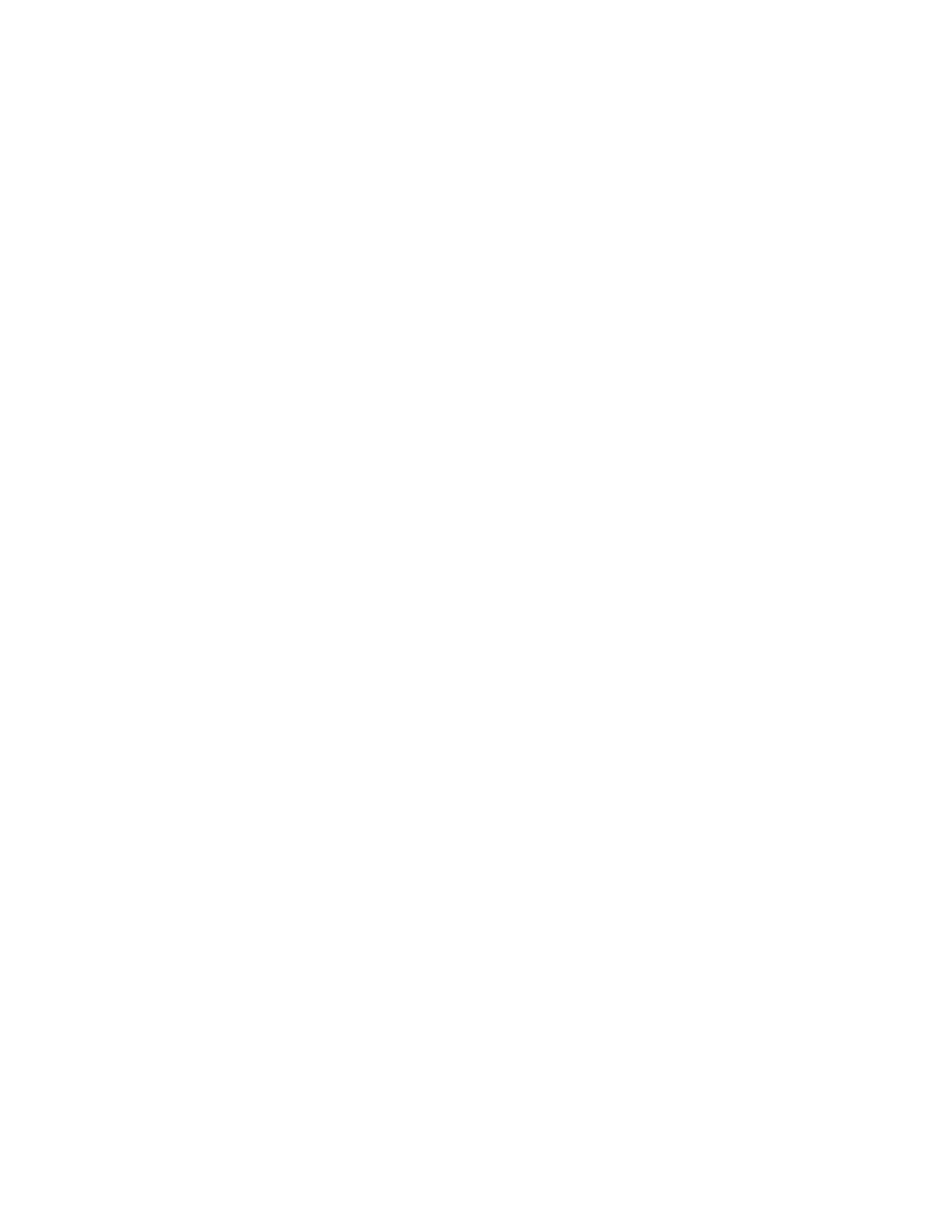
v
Regina, Valéria, Helen, Melissa, Raquel, Alice, Carol Berra, Carol Quayle, Maria
Helena, Marinalva, Tatiana, Helotônio e Renata. A amizade desenvolvida com essas
pessoas, em tão pouco tempo, foi impressionante. São pessoas que ajudaram não somente
na minha formação acadêmica, me passando conhecimento, mas também trouxeram-me
um grande aprendizado pessoal.
Ao Fábio e a Adriana, pela disponibilidade e ajuda em muitas etapas desse
trabalho. Esses agradecimentos estendem-se ao Hospital de Caridade de Ijuí.
A Fernanda Dondé, “minha” primeira aluna de iniciação científica. Pela amizade,
companherismo e pela grande ajuda prestada durante esse período. Estou crescendo
muito com essa orientação. Acredito que estou aprendendo mais do que ensinando.
Aos meus GRANDES amigos: Tomaz, Rovian, Cássio e Gustavo. Todos vocês
foram peças fundamentais para eu alcançar esse objetivo. Grandes noites de conversas,
churrasco e, claro, aquela cervejinha gelada. Sempre que precisei, vocês estavam lá,
prontos para ajudar, o meu muito obrigado por tudo.
Um parágrafo especial ao meu melhor amigo, meu irmão André. O agradecimento
a essa pessoa torna-se complicado, pois faltariam páginas para descrever tamanha
gratidão a ele. André, meu muito obrigado por tudo que você já fez por mim, e ainda,
pela grande ajuda que você me deu nesse mestrado. Você e eu sabemos que, sem tua
ajuda, eu não teria começado, e muito menos terminado esse mestrado.
Aos meus pais, Armando e Clereci. Inicialmente pessoas que me incetivaram a
lutar e buscar meus sonhos. Forneceram-me o combustível fundamental para isso, o
amor. Agora, terminanda mais essa etapa de minha vida, reconheço que a educação
fornecida por vocês, bem como o apoio dado em todos os momentos, foram cruciais para
a realização desse meu projeto. Muito obrigado, esse título também é de vocês.
A Fernanda Bianchini, uma pessoa que sempre esteve no meu lado, fornecendo-
me carinho e respeito. Meu muito obrigado pela confiança, e claro, pela paciência que
você teve durante todo esse período.
A todos que de alguma forma colaboraram com o desenvolvimento desse
trabalho.
Obrigado.