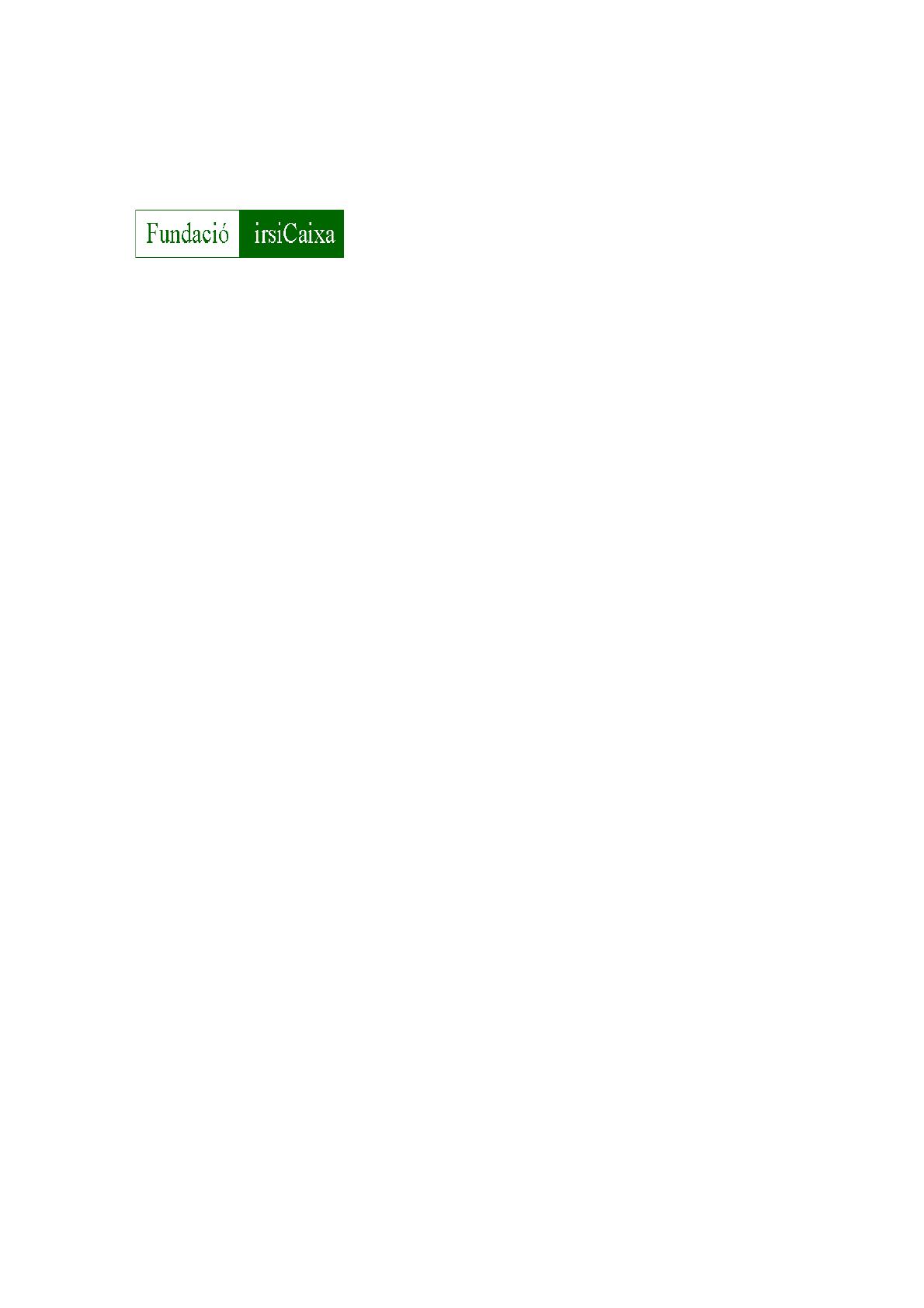
El Dr. Javier Martínez-Picado y la Dra. Lidia Ruiz Tabuenca investigadores
del Laboratorio de Retrovirología del Hospital Germans Tiras i Pujol (Fun-
dación IrsiCaixa, Institut de Recerca de la SIDA de “la Caixa”)
HACEN CONSTAR:
Que el trabajo experimental y la redacción de la tesis titulada: “
Variaciones
en el Fitness del VIH-1 durante la Terapia Antirretroviral
“ ha sido realiza-
da por Julia García Prado y consideran que es apta para su lectura y defen-
sa ante un tribunal de tesis, y con ello, optar al grado de Doctora por la Uni-
versidad Autónoma de Barcelona.
Para que haga constancia en este documento en Badalona, 6 de Junio del
2005
Dr. Javier Martínez-Picado Dra. Lidia Ruiz Tabuenca
Investigador Investigadora
Fundación IrsiCaixa Fundación IrsiCaixa