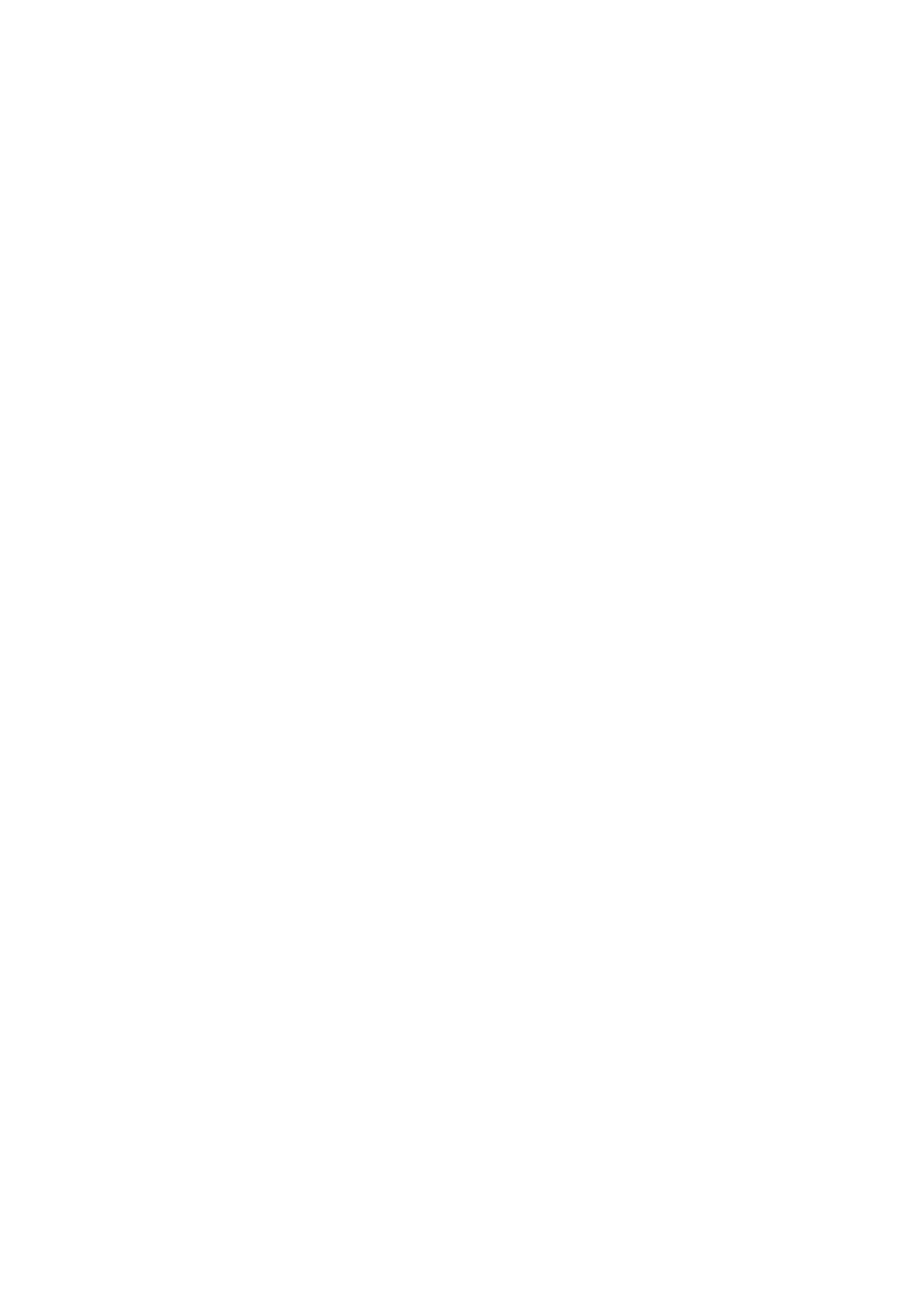
105
________________
RESULTATS
1998). Cationic lipids (Li and Ma, 2001; Mountain, 2000) and synthetic peptides
(Sparrow et al., 1998) have been explored as coating devices for expressible DNA,
and multifunctional proteins for cell targeted DNA delivery have been constructed
by combining bioactive protein domains from different origins (Arís et al., 2000;
Fominaya and Wels, 1996; Paul et al., 1997). These independent elements can supply
DNA-condensing, cell binding, cell internalization, endosome-disrupting and
nuclear targeting activities to the resulting vehicles without most of the
inconveniences of potentially infective material (Navarro et al., 1998). The intrinsic
flexibility of these vectors offers wider perspectives for the generation of promising
vehicle prototypes for specific therapeutical needs.
For the central nervous system (CNS) the value of these constructs is of special
relevance, as DNA delivery by different viral species has been achieved (Brooks et
al., 2002; Costantini et al., 2000; Dewey et al., 1999; Sapolsky and Steinberg, 1999),
but accompanied by unacceptable toxicity (Nilaver et al., 1995), persistent
inflammation (Dewey et al., 1999), immune activation (Byrnes et al., 1996; Wood et
al., 1996), and demyelination (Byrnes et al., 1996; Nilaver et al., 1995). In addition,
viral delivery has been shown to potentially break tolerance to endogenous proteins
(Zinkernagel et al., 1990). Likewise, the peripheral re-administration of viral vectors
to animals previously injected intracerebrally with the same vectors induces a
delayed-type hypersensitivity reaction in the neural parenchyma (Byrnes et al.,
1996).
Alternative gene delivery strategies for the CNS, such as intracerebral injection of
polyethylenimine/DNA (Bousiff et al., 1995) or lipid/DNA complexes (Hecker et
al., 2001; Shi and W.M., 2000) have been explored, although they still need further
improvement (Li and Ma, 2001). In this regard, we have previously reported the
construction of 249AL, a non-viral vector based on an engineered β-galactosidase
protein (Arís et al., 2000). Taking advantage of β-galactosidase nuclear localization
motifs (McInnis et al., 1995), two additional functional domains were introduced
into this bacterial protein. An Arg-Gly-Asp (RGD) integrin-binding and cell
internalization peptide and a polylysine tail with DNA condensing properties,
rendered this multifunctional protein an efficient vector for gene delivery into
cultured cells (Arís et al., 2000).
In this study, we have characterized 249AL as a vector for in vivo gene delivery to
the intact and damaged postnatal rat CNS. Gene therapy attempts of damaged
brains would be of special relevance for the therapeutic approches of perinatal
hypoxic-ischemic insult (or related pathologies), involving severe neurological
consequences as spastic paresis, choreo-atheosis, ataxia, disorders of sensomotor
coordination, or impairment of intellectual ability (Berger and Garnier, 1999), and
with a significant incidence in human populations (Ohrt et al, 1995). In this regard,
excitotoxicity by NMDA receptor activation has been largely used as a model for
hypoxic-ischemic injury to the postnatal brain (Ikonomidou et al, 1989; Olney 1990).