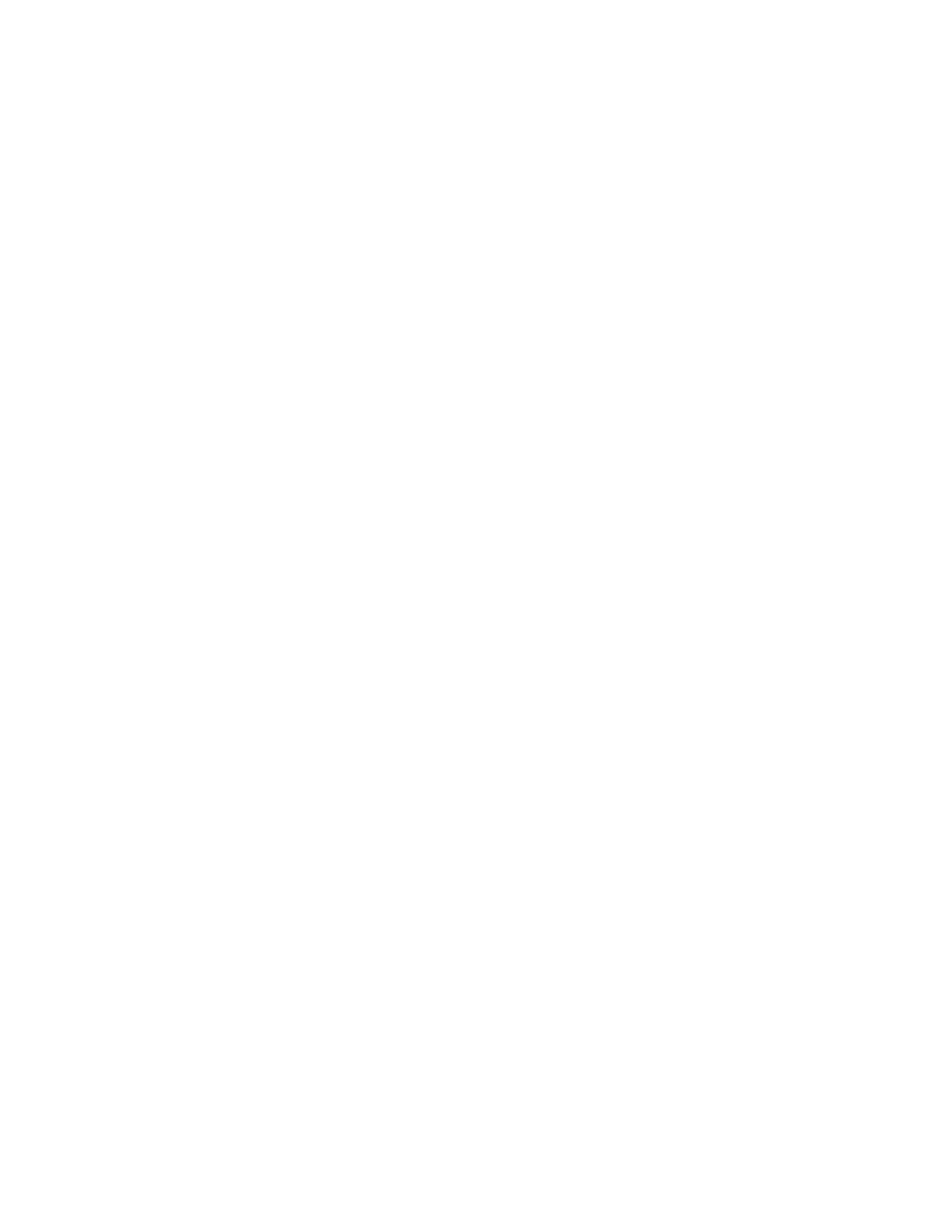
iii
SOMMAIRE
Un des défis les plus pressants offerts aux écologistes des communautés actuellement consiste
à prédire la réponse des assemblages d'espèces au changement environnemental global. Les
études départageant la contribution de la variation observée à l'intérieur des espèces et entre
les espèces à la variation fonctionnelle observée entre les communautés le long de gradients
environnementaux spatiaux sont particulièrement utiles à cette fin. Elles permettent en effet
d'évaluer la part que l'adaptation locale et la plasticité phénotypique (variation
intraspécifique), en plus du renouvellement en espèces (variation interspécifique), pourraient
jouer dans la médiation des changements environnementaux par les communautés végétales.
Ce mémoire vise à évaluer la contribution de la variation intraspécifique au renouvellement en
traits entre des communautés végétales le long d'un flanc de montagne, ainsi que les contextes
environnementaux et écologiques dans lesquels une plus grande ou plus faible contribution de
cette variation intraspécifique pourrait être attendue. Pour ce faire, j’ai quantifié la variation
dans trois traits fonctionnels distincts (phénologie florale, surface foliaire spécifique et
hauteur) entre, et à l'intérieur de 51 espèces végétales herbacées de sous-bois distribuées entre
des communautés couvrant une transition de la forêt de feuillus à la forêt boréale, au Parc
national du Mont-Mégantic (Qc). Je présente à la fois un nouveau cadre conceptuel établissant
des hypothèses et prédictions sur les contextes environnementaux et écologiques pouvant
déterminer la magnitude de la contribution de la variation intraspécifique à l'association trait-
environnement entre les communautés végétales, que je teste à l'aide de ce jeu de données.
Je rapporte que l'importance relative de la variation intraspécifique au renouvellement
fonctionnel entre les communautés est dépendante de l'axe environnemental considéré, étant la
plus forte le long d'axes non-climatiques, soit l'ouverture de la canopée et l'acidité du sol. Cette
contribution est également déterminée de façon importante par la structure des réponses des
espèces au gradient, en particulier par la direction et la magnitude de la variation