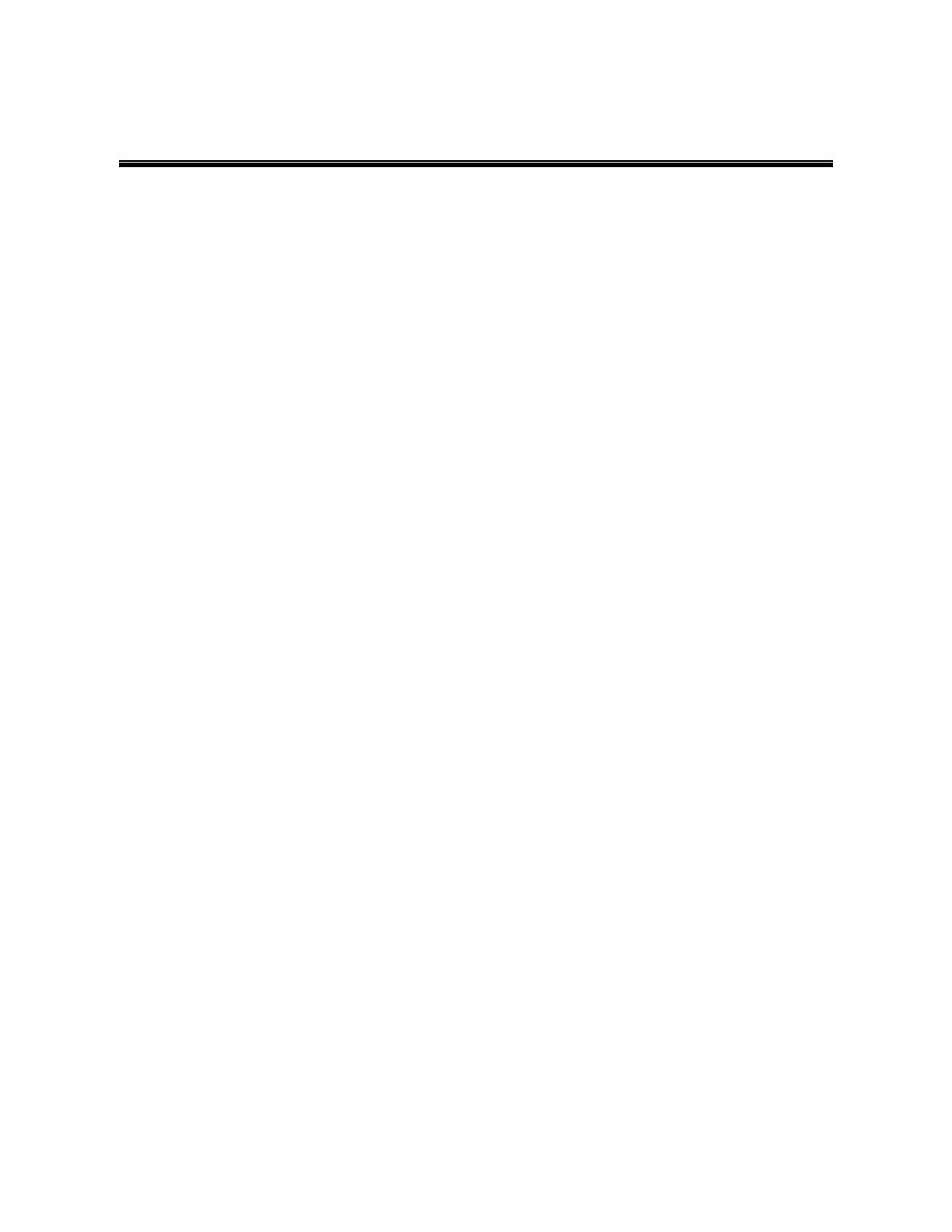
i
Résumé
Dans cette thèse, l’impact du polymorphisme rs3846662 sur l’épissage alternatif de la
3-hydroxy-3-méthylglutaryl coenzyme A réductase (HMGCR) a été investigué in vivo, chez
des patients atteints d’hypercholestérolémie familiale (HF) ou de maladie d’Alzheimer (MA).
Le premier manuscrit adresse la problématique de la normalisation de la quantification relative
des ARNm par PCR quantitative. Les découvertes présentées dans ce manuscrit nous ont
permis de déterminer avec un haut niveau de confiance les gènes de référence à utiliser pour la
quantification relative des niveaux d’ARNm de l’HMGCR dans des échantillons de sang
(troisième manuscrit) et de tissus cérébraux post-mortem (quatrième manuscrit).
Dans le deuxième manuscrit, nous démontrons grâce à l’emploi de trois cohortes de
patients distinctes, soit la population canadienne française du Québec et les deux populations
nord américaines « Alzheimer’s Disease Cooperative Study (ADCS) » et « Alzheimer’s
Disease Neuroimaging Initiative (ADNI) », que le génotype AA au locus rs3846662 confère à
ces porteurs une protection considérable contre la MA. Les femmes porteuses de ce génotype
voient leur risque de MA diminuer de près de 50% et l’âge d’apparition de leurs premiers
symptômes retarder de 3.6 ans. Les porteurs de l’allèle à risque APOE4 voient pour leur part
leurs niveaux de plaques séniles et dégénérescences neurofibrillaires diminuer
significativement en présence du génotype AA. Enfin, les individus atteints de déficit cognitif
léger et porteurs à la fois de l’allèle APOE4 et du génotype protecteur AA voient leur risque
de convertir vers la MA chuter de 76 à 27%.
Dans le troisième manuscrit, nous constatons que les individus atteints d’HF et
porteurs du génotype AA ont, contrairement au modèle établi chez les gens normaux, des
niveaux plus élevés de cholestérol total et de LDL-C avant traitement comparativement aux
porteurs de l’allèle G. Le fait que cette association n’est observée que chez les non porteurs de
l’APOE4 et que les femmes porteuses du génotype AA présentent à la fois une augmentation
des niveaux d’ARNm totaux et une résistance aux traitements par statines, nous indique que ce