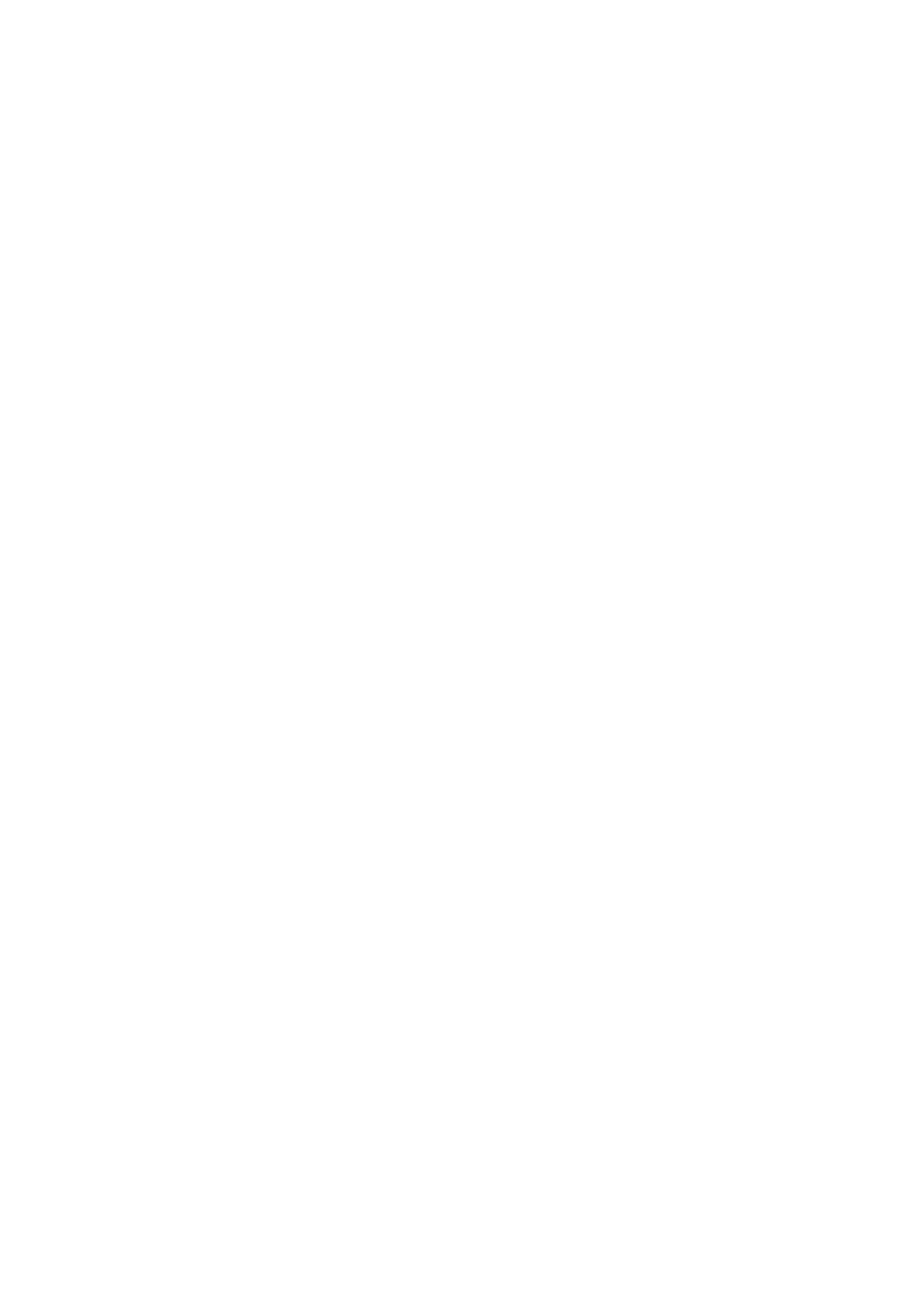
IV
Mais ue seait l’uipe « écotox » sans ce cher Abderhamane ?! Merci à toi, pour ton aide dans la
réalisation de ce travail mais aussi pour toutes les discussions scientifiques, politiques, etc.
Mei à toi aussi, Isaelle pou ’aoi tasis to saoi otaet pou alise les dissections
de poissons, mais aussi et surtout pour les bons moments partagés ensemble ! Mei de ’aoi
aueilli au sei de ta faille. Mei à ous, Gad, Tagu, Ge et toi aussi Maël pou ui j’ai ue
pensée, pour votre chaleureux accueil.
Un énorme merci à vous mes amis de galère ! Vous les thsads ue j’ai eu la hae et l’hoeu de
côtoyer ! Le futur de la recherche ! Merci à mes collègues féminines du bureau de thésard de MMS,
Marie (ou plutôt Marie Gaga), Elodie (ma petite blanchette), Marieke (la princesse de la mer) mais
aussi à mes collègues « écotox » Pierre-Emmanuel (PE) et Olivia! Et également à Thomas et
Guillaue ui ’ot appot tepoaieet u soutie asuli das le ueau ! On a partagé de
super moment ! Entre les apéros labo, les sorties escalades, les soirées vin/fromage, Vieux Bouc Eau
s’est od !), les discussions philosophiques ou pas, les mots croisés, la minute cucuculturelle
uotidiee… Mei pou tout ça !
Merci également aux thésards MMSiens des étages « supérieurs », Nuria, Sigrid, Anthony, Mathilde
et Ismaïl pour les discussions de couloir, de laboratoire, vos conseils et votre soutiens !
Et non, je ne vous oublie pas les petites nouvelles du bureau ! Ana Camila Dos Santos Dias De La
Playa Del Sol et Anne-Isaline Van Bohemen. Les sportives du labo ! Merci à toutes les deux ! Je vous
souhaite une bonne thèse ! Profitez bien de ces moments ! et merci pour votre soutien !
Merci à Claude Amiard-Tiuet et Jea Claude Aiad de ’aoi peis de patiipe à u ouage
scientifique mais aussi pour les discussions scientifiques et rugbystiques !
U gad ei à toute l’uipe MMS ! Pour votre accueil au sein du laboratoire. Pour les bons
moments partagés qui rendent le travail moins dur ! Je vous souhaite à tous du succès dans vos
recherches ! Je eeie galeet les ees de l’uipe spotie MMS ae ui j’ai patag de
nombreuses défaites aux rencontres avec les étudiants. Je comptes sur vous pour perpétuer cette
tradition !
Merci à mes collègues de mon second laboratoire le LABERCA pour leur accueil et leur aide dans la
réalisation de ce travail.
Mei au ees de l’Istitut d’Études Eioeetales d’Asteda. [Attetio les eu je
e lae das des phases e aglais…] I would like to express my special thanks to my colleagues