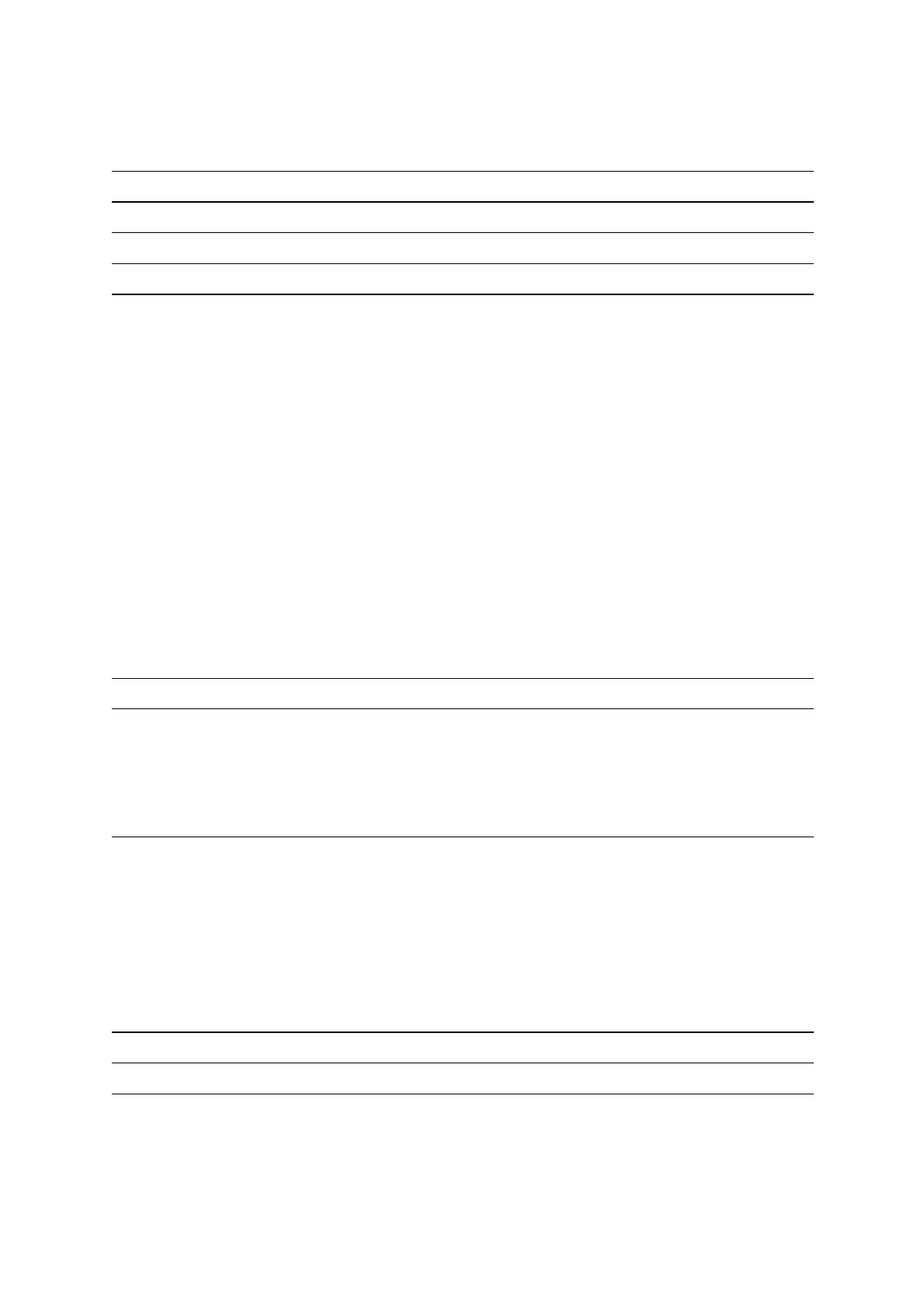
5
SOMMAIRE
REMERCIEMENTS ............................................................................................................... 2
RESUME .................................................................................................................................. 3
ABREVIATIONS ..................................................................................................................... 4
SOMMAIRE ............................................................................................................................. 5
INTRODUCTION.................................................................................................................... 6
1 La présence d’un danger : une condition nécessaire à l’activation du système immunitaire ?
............................................................................................................................................................ 6
1.1 La théorie du danger et les signaux de danger ................................................................................... 6
1.2 Les réponses immunitaires au danger ................................................................................................. 9
2 Les réponses immunitaires naturelles dans le cancer ............................................................... 19
2.1 Les tumeurs : tolérance ou immunité ? ............................................................................................. 19
2.2 Environnement tumoral et immunosuppression .............................................................................. 31
3 Les solutions thérapeutiques pour activer des réponses immunitaires anti-tumorales
efficaces ............................................................................................................................................ 39
3.1 L’utilisation de ligands de TLR en immunothérapie anti-cancéreuse ........................................... 39
3.2 Effets immunostimulants des thérapies anti-cancéreuses conventionnelles ................................... 43
4 Contexte d’étude et objectifs de la thèse .................................................................................... 51
4.1 Les mécanismes moléculaires de l’immunogénicité de la mort cellulaire induite par certaines
thérapies anti-cancéreuses conventionnelles ........................................................................................... 51
4.2 Problématiques de la thèse ................................................................................................................. 53
MATERIEL ET METHODES ............................................................................................. 54
RESULTATS .......................................................................................................................... 57
1 Rôle de l’activation de l’inflammasome NLRP3 et de la production d’IL-1β par les cellules
dendritiques dans l’efficacité des chimiothérapies immunogènes .............................................. 57
2 Rôle des LTγδ producteurs d’IL-17 dans l’efficacité thérapeutique de thérapies anti-
cancéreuses conventionelles ........................................................................................................... 60
DISCUSSION ......................................................................................................................... 62
1 Importance des modèles de tumeurs établies ............................................................................ 62
2 Rôle de l’ATP extra-cellulaire dans le développement de réponses immunitaires spécifiques
.......................................................................................................................................................... 63
3 Rôle de l’IL-1β dans le développement de réponses Th1 et Tc1 spécifiques .......................... 65
4 Rôle de l’IL-17 dans le développement de réponses Th1 et Tc1 spécifiques .......................... 66
5 Rôle de l’IL-17 et de l’IL-1β dans la progression tumorale ..................................................... 69
CONCLUSION ET PERSPECTIVES ................................................................................. 70
REFERENCES ....................................................................................................................... 73
ANNEXES .............................................................................................................................. 88