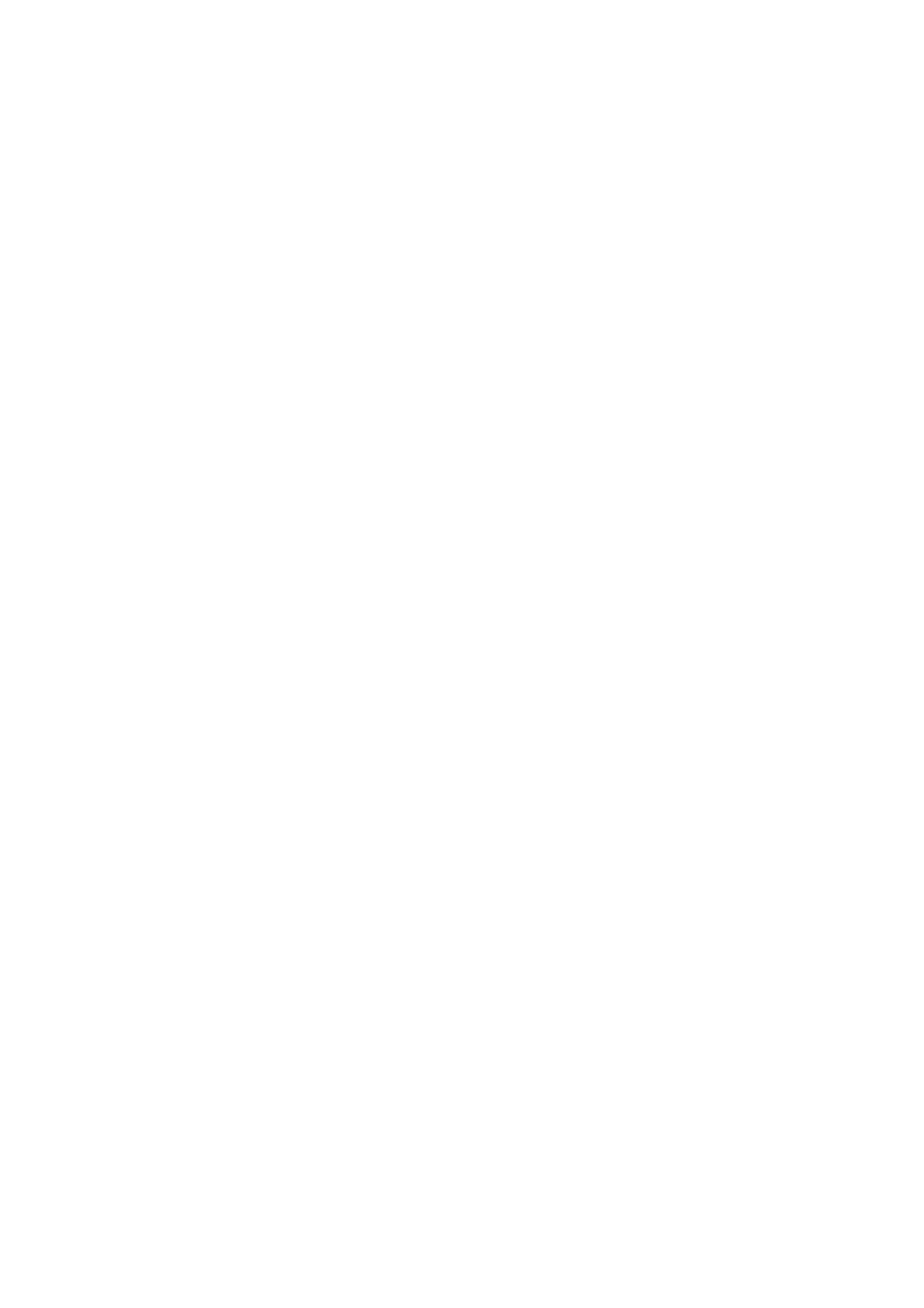
LISTE DES ABRÉVIATIONS
AID : « activation-induced cytidine deaminase »
aa : acide aminé
Ac : anticorps
ADNc : acide désoxyribonucléique complémentaire
Ag : antigène
AP1 : « activator protein 1 »
ARN : acide ribonucléique
ATL : « adult T-cell leukemia/lymphoma »
ATM : « ataxia telangiectasia mutated »
BARTs : « BamA rightward transcripts »
BCR : « B-cell receptor »
BLIMP : « B lymphocyte-induced maturation protein »
CBD : « cytokine binding domain »
CD : « cluster of differentiation »
CG : centre germinatif
CLC : « cardiotrophin-like cytokine »
CLF : « cytokine-like factor »
CLMF : « cytotoxic lymphocyte maturation factor »
CNTF(R) : « ciliary neurotrophic factor (receptor) »
CREB : « cyclic AMP-responsive element-binding protein »
CRTH2 : « chemoattractant receptor-homologous molecule expressed on Th2 cells »
CT : « cardiotrophin »
CTAR : « C-terminal activating region »
EBER : « Epstein-Barr virus small RNA »
EBI3 : « EBV-induced gene 3 »
EBNA : « EBV nuclear antigen »
EBV : « Epstein-Barr virus »
ELISA : « enzyme-linked immunosorbent assay »
FGFR : « fibroblast growth factor receptor »
G-CSF : « granulocyte colony-stimulating factor »
GM-CSF : « granulocyte-macrophage colony-stimulating factor »
HAM : « HTLV-1-associated myelopathy »
HTLV-1 : « Human T-cell leukemia virus type 1 »
HRS : Hodgkin and Reed-Sternberg
IFN : interféron
Ig : immunoglobuline
IL : interleukine
IP-10 : « gamma interferon-inducible protein 10 »
IRF4 : « interferon regulatory factor »
Jak : Janus kinase
JNK : « c-jun N-terminal kinase »
kDa : kiloDalton
KO : « knock out »
LIF : « leukemia inhibitory factor »
LLC : leucémie lymphoïde chronique
LMP : « latent membrane protein »
LPS : lipopolysaccharide
LTR : « long terminal repeat »