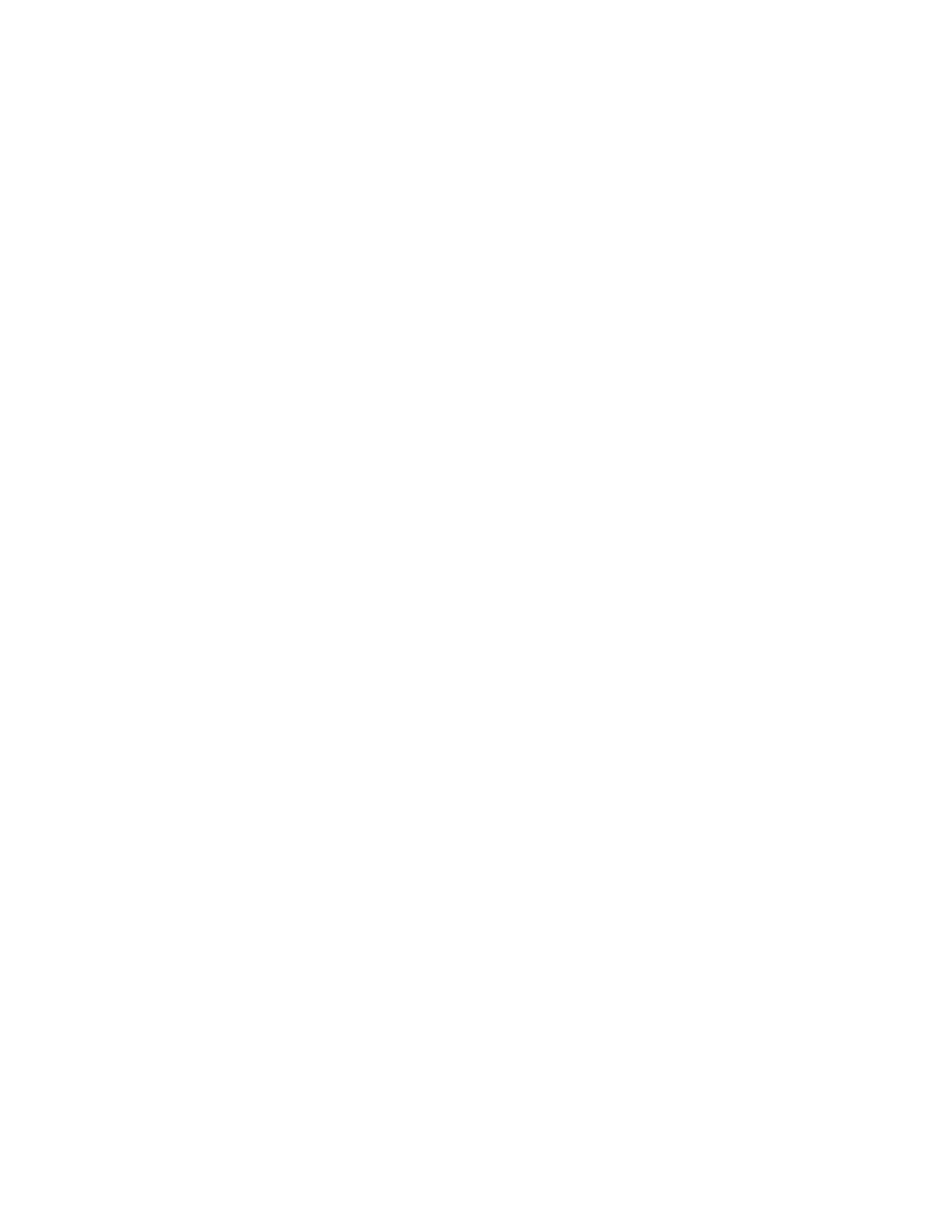
Résumé
La candidose oro-pharyngée (COP) est l’infection fongique opportuniste la plus
commune chez les individus infectés par le VIH-1. La production des cytokines Il-17 et Il-22
par les lymphocytes Th17 est importante lors de la résolution de la COP, puisque ces
cytokines induisent la production de peptides antifongiques et le recrutement des neutrophiles
polymorphonucléaires. Toutefois, les lymphocytes Th17 sont préférentiellement déplétés chez
les individus infectés par le VIH-1. Le modèle de COP chez la souris transgénique (Tg)
CD4C/HIVMutA, exprimant les gènes nef, env et rev du VIH-1, permettra de déterminer si des
altérations quantitatives et/ou fonctionnelles des sous-populations de lymphocytes T CD4+
causent la sensibilité à la candidose. Les sous-populations Th1, Th2, Th1Th17, Th17 et Treg,
ainsi que leurs précurseurs, les lymphocytes T CD4+ naïfs, sont sévèrement déplétées dans les
ganglions cervicaux de la souris Tg. Cependant, les lymphocytes T CD4+ naïfs conservent la
capacité à se différencier in vitro en présence de cytokines polarisantes et à produire les
cytokines typiques des diverses sous-populations. De plus, les cytokines requises pour la
polarisation des lymphocytes T CD4+ naïfs n’étaient pas réduites dans les ganglions cervicaux
des souris Tg, 7 jours après le début de l’infection. Les gènes S100a8, Ccl20, Il17 et Il22
étaient surexprimés en réponse à la COP chez la souris non-Tg, mais pas chez la souris Tg. Le
traitement de souris Tg infectées à l’aide de la combinaison des cytokines Il-17 et Il-22 réduit
significativement la charge fongique buccale de C. albicans et le nombre d’hyphes dans
l’épithélium de la langue et restaure la capacité à surexprimer des gènes S100a8, Ccl20 et Il22.
Ces résultats démontrent que la perturbation de l’induction de l’immunité innée par l’Il-17 et
l’Il-22 augmente la susceptibilité à la COP chez la souris Tg.
Mots-clés: Candidose oro-pharyngée, cytokine, Il-17, Il-22, modèle animal, Th17, lymphocyte
T régulateur, VIH-1.