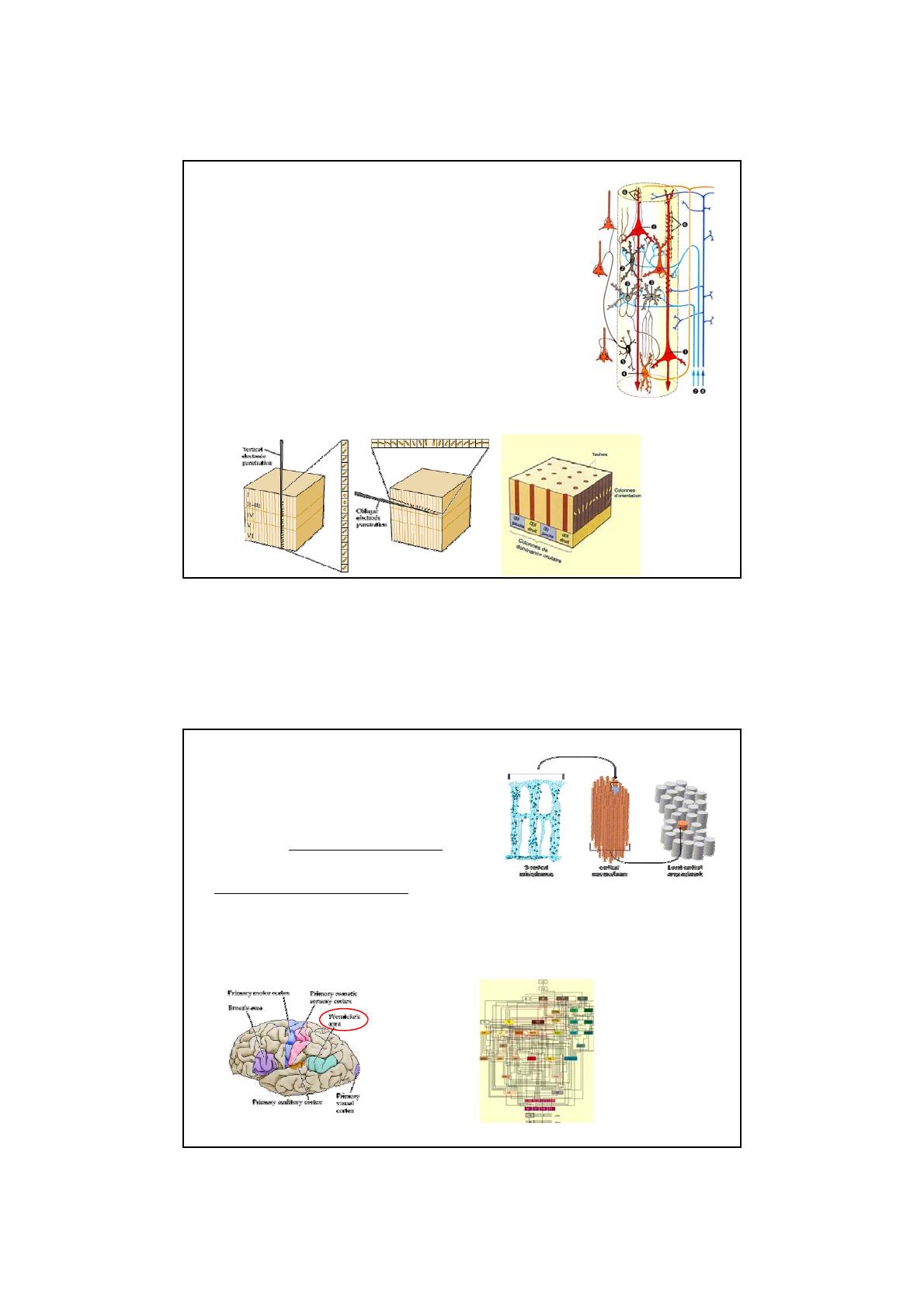
19/10/2009
5
2.Introductiontoneuronalnetworks
•Corticalneurons haveanhorizontalandverticalorganization:they
areorganized in layers andinminicolumns .
•Aminicolumn is abasiccorticalnetworkcontaining 100‐200
neurons (excitatory andinhibitory,localandprojecting)="Building
block"formorespecialized,morediversecorticalmacrocolumns
•Minicolomns organize inmacrocolumns:50‐100minicolumns.
Neurons inthesame macrocolumn share functional properties
e.g.inprimary cortices:
‐Neurons inamacrocolumn respond tosimilar stimulus
localizations andproperties
‐Neighboring macrocolumns "represent"neighboring regions of
theperiphery (topological correspondence)
Thus,corticalneurons organize innetworksat different scales:
‐Corticalminicolumns
‐Corticalmacrocolumns
‐Localcorticalareanetworks
‐Andfinally inlarge‐scale neuronalnetworks
•Finally,large‐scale neuronalnetworksaremadeupfrom several localcorticalareanetworks
interconnected bylong‐rangeprojections
•Function together inallcorticalfunctions
•Thenetworking ofdiscrete corticalareasinto large‐scale networksis dynamic (e.g.understanding
written andspoken language engagepartially overlapping networks)
Completevisualnetworksinthemacaquebrain(FellemanandVanEssen,1990)Simplifiedlanguagenetwork