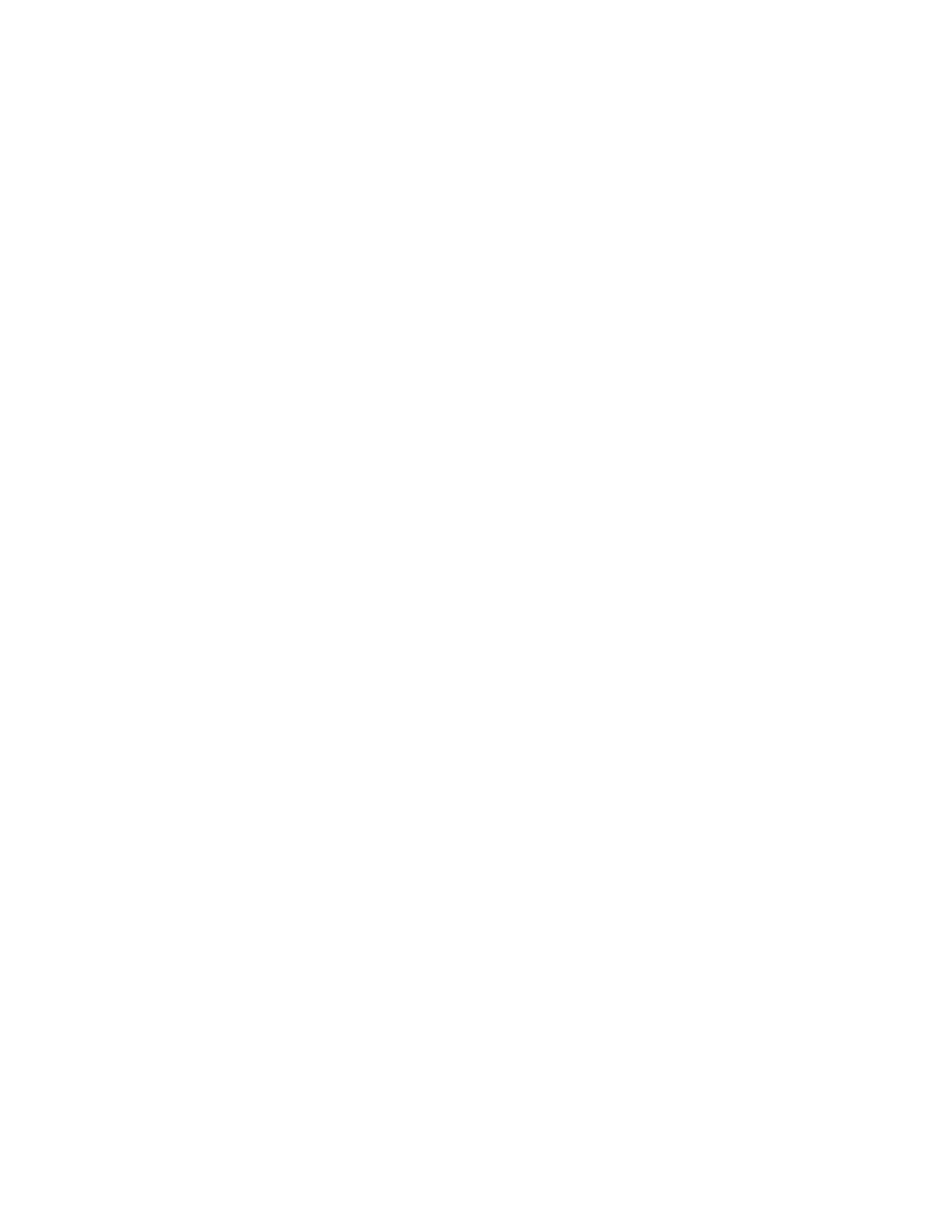
i
RÉSUMÉ
Alors que d’énormes efforts sont mis de l’avant pour mettre en place des stratégies
thérapeutiques contre l’infection au VIH-1, il est nécessaire de mieux cerner les déterminants
viraux qui aideront à l’efficacité de celles-ci. En ce sens, une volumineuse littérature
scientifique suggère que les anticorps contre le VIH-1 possédant une capacité à induire une
réponse effectrice dépendante de leur portion Fc puissent jouer un rôle important dans la
prévention de l’infection et dans la progression de la maladie. Cependant, peu d’information
est disponible concernant les déterminants reconnus par ces anticorps et comment le virus s’en
protège. Le but des travaux présentés dans cette thèse est donc d’élucider les mécanismes
viraux contrôlant la reconnaissance des cellules infectées par ces anticorps capables d’induire
une réponse effectrice. De par les corrélats de protection identifiés au cours de l’essai vaccinal
RV144, les travaux présentés ici se concentrent sur la réponse cytotoxique dépendante des
anticorps (ADCC), puisqu’il s’agit d’une réponse effectrice suggérée pour avoir joué un rôle
dans la protection observée dans le RV144, seul essai vaccinal anti-VIH à avoir démontré un
certain degré de protection. De plus, plusieurs anticorps capables d’induire cette réponse
contre le VIH sont connus pour reconnaître les glycoprotéines de surface du virus (Env) dans
une conformation dite ouverte, c’est-à-dire la conformation adoptée lors de la liaison d’Env
avec son récepteur CD4 (épitopes CD4i). Nous avons mis au point deux techniques in vitro
permettant d’étudier ces changements de conformation ainsi que leur impact sur la réponse
ADCC.
Les techniques mises au point, un ÉLISA sur base cellulaire pour mesurer les
changements de conformation d’Env ainsi que la mesure de la réponse ADCC par cytométrie
en flux, nous ont permis de démontrer comment le virus empêche l’exposition des épitopes
d’Env CD4i. L’activité simultanée des protéines accessoires virales Nef et Vpu sur le retrait
du récepteur CD4 de la surface des cellules infectées et l’inhibition du facteur de restriction
Tétherine / BST-2 par Vpu contrôlent à la fois les niveaux d’Env et de CD4 à la surface
cellulaire et donc modulent l’interaction Env-CD4 et ultimement la susceptibilité à la réponse
ADCC contre les épitopes CD4i reconnus par des anticorps hautement prévalents lors de
l’infection au VIH. Également, nous démontrons comment de petits composés mimant la