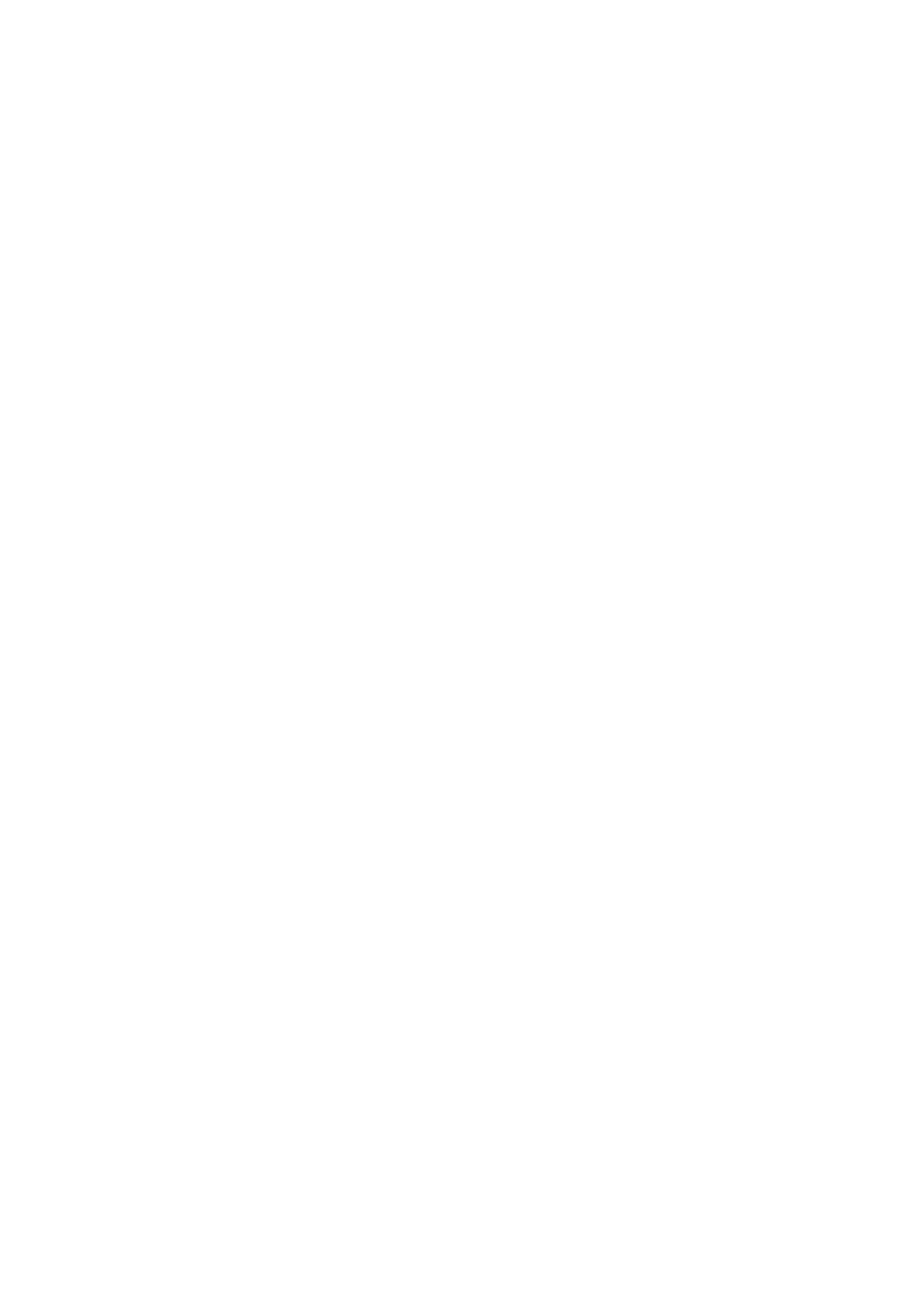
5
a)
La molécule CD3
..............................................................................................................31
b)
La molécule CD2
..............................................................................................................33
c)
La molécule CD4
..............................................................................................................33
2. Campath-1H......................................................................................................................34
3. Sérum anti-lymphocytaire............................................................................................35
C. Mise en place d’un chimérisme ...................................................................................36
1. Définitions et mécanismes ............................................................................................36
2. Protocoles d’induction de tolérance par induction d’un chimérisme...............38
III. La tolérance « opérationnelle » .............................................................................. 42
IV. Les cellules T régulatrices humaines .....................................................................44
A. Les cellules T CD4+.........................................................................................................46
1. Les cellules T CD4+CD25+ naturelles........................................................................46
a)
Origine et localisation
....................................................................................................46
b)
Caractéristiques phénotypiques
...................................................................................47
c)
Caractéristiques fonctionnelles
....................................................................................49
d)
Cellules T CD4+CD25+ régulatrices et pathologies
...............................................50
2. Les cellules T CD4+ régulatrices induites.................................................................52
a)
Les lymphocytes Tr1
.......................................................................................................52
b)
Les lymphocytes Th3
......................................................................................................54
B. Les cellules T CD8+ suppressives ................................................................................54
1. Les cellules T CD8+ suppressives (Ts) de type 1 .....................................................55
2. Les cellules T CD8+ suppressives de type 2..............................................................57
3. Les cellules T CD8+ suppressives de type 3..............................................................57
C. Les cellules NKT ..............................................................................................................58
D. Les cellules T anti-idiotypiques et anti-ergotypiques............................................59
V. Les glucocorticoïdes (GC) ....................................................................................62
A. Historique des corticostéroïdes....................................................................................62
B. Les hormones stéroïdiennes .........................................................................................63
C. Les glucocorticoïdes et leurs récepteurs....................................................................63
D. Propriétés anti-inflammatoires et immunosuppressives des glucocorticoïdes64
1. Glucocorticoïdes et voies de signalisations intracellulaires.................................65
2. GC et apoptose..................................................................................................................68