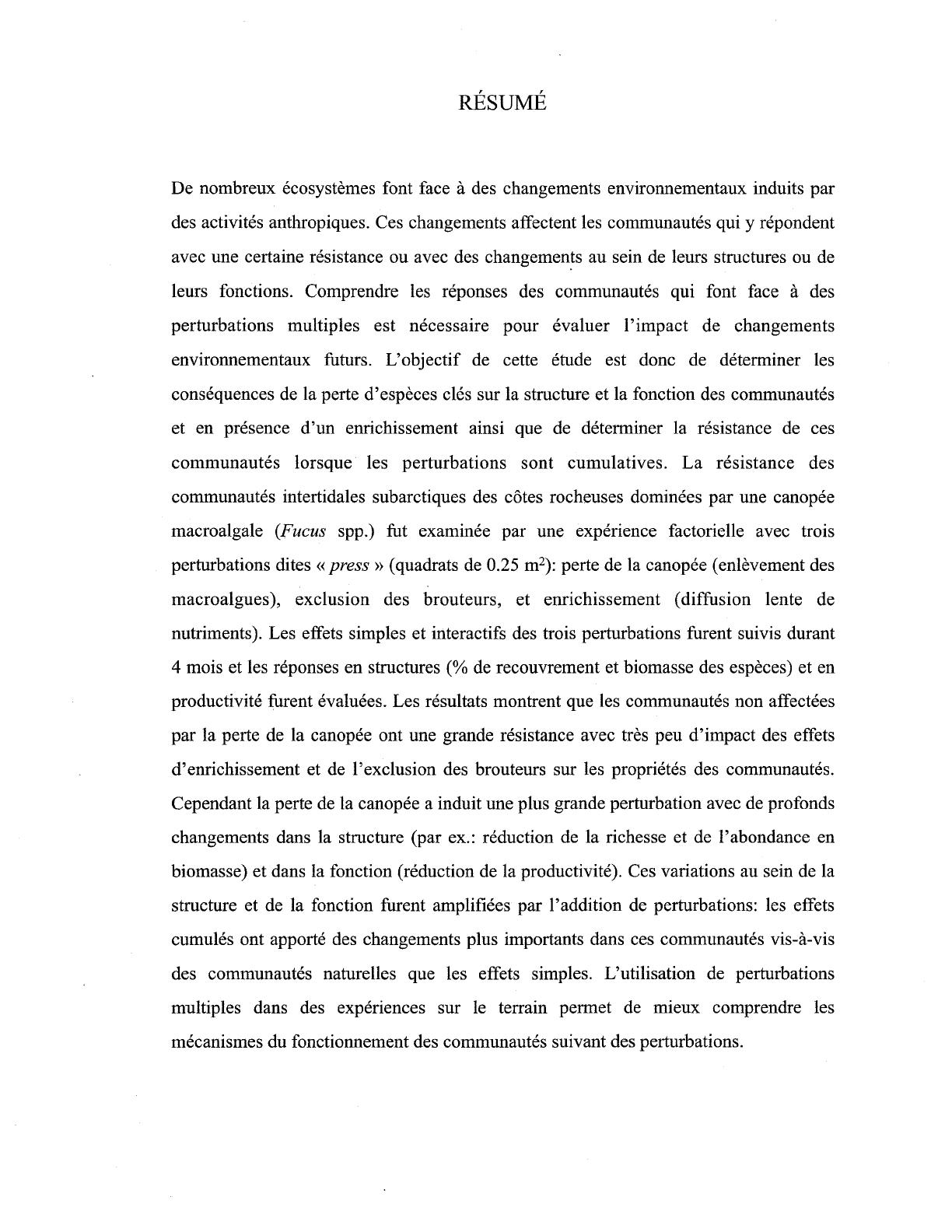
RESUME
De nombreux écosystèmes font face à des changements environnementaux induits par
des activités anthropiques. Ces changements affectent les communautés qui y répondent
avec une certaine résistance ou avec des changements au sein de leurs structures ou de
leurs fonctions. Comprendre les réponses des communautés qui font face à des
perturbations multiples est nécessaire pour évaluer l'impact de changements
environnementaux futurs. L'objectif de cette étude est donc de déterminer les
conséquences de la perte d'espèces clés sur la structure et la fonction des communautés
et en présence d'un enrichissement ainsi que de déterminer la résistance de ces
communautés lorsque les perturbations sont cumulatives. La résistance des
communautés intertidales subarctiques des côtes rocheuses dominées par une canopée
macroalgale {Fucus spp.) fut examinée par une expérience factorielle avec trois
perturbations dites «press » (quadrats de 0.25 m2): perte de la canopée (enlèvement des
macroalgues), exclusion des brouteurs, et enrichissement (diffusion lente de
nutriments). Les effets simples et interactifs des trois perturbations furent suivis durant
4 mois et les réponses en structures (% de recouvrement et biomasse des espèces) et en
productivité furent évaluées. Les résultats montrent que les communautés non affectées
par la perte de la canopée ont une grande résistance avec très peu d'impact des effets
d'enrichissement et de l'exclusion des brouteurs sur les propriétés des communautés.
Cependant la perte de la canopée a induit une plus grande perturbation avec de profonds
changements dans la structure (par ex.: réduction de la richesse et de l'abondance en
biomasse) et dans la fonction (réduction de la productivité). Ces variations au sein de la
structure et de la fonction furent amplifiées par l'addition de perturbations: les effets
cumulés ont apporté des changements plus importants dans ces communautés vis-à-vis
des communautés naturelles que les effets simples. L'utilisation de perturbations
multiples dans des expériences sur le terrain permet de mieux comprendre les
mécanismes du fonctionnement des communautés suivant des perturbations.