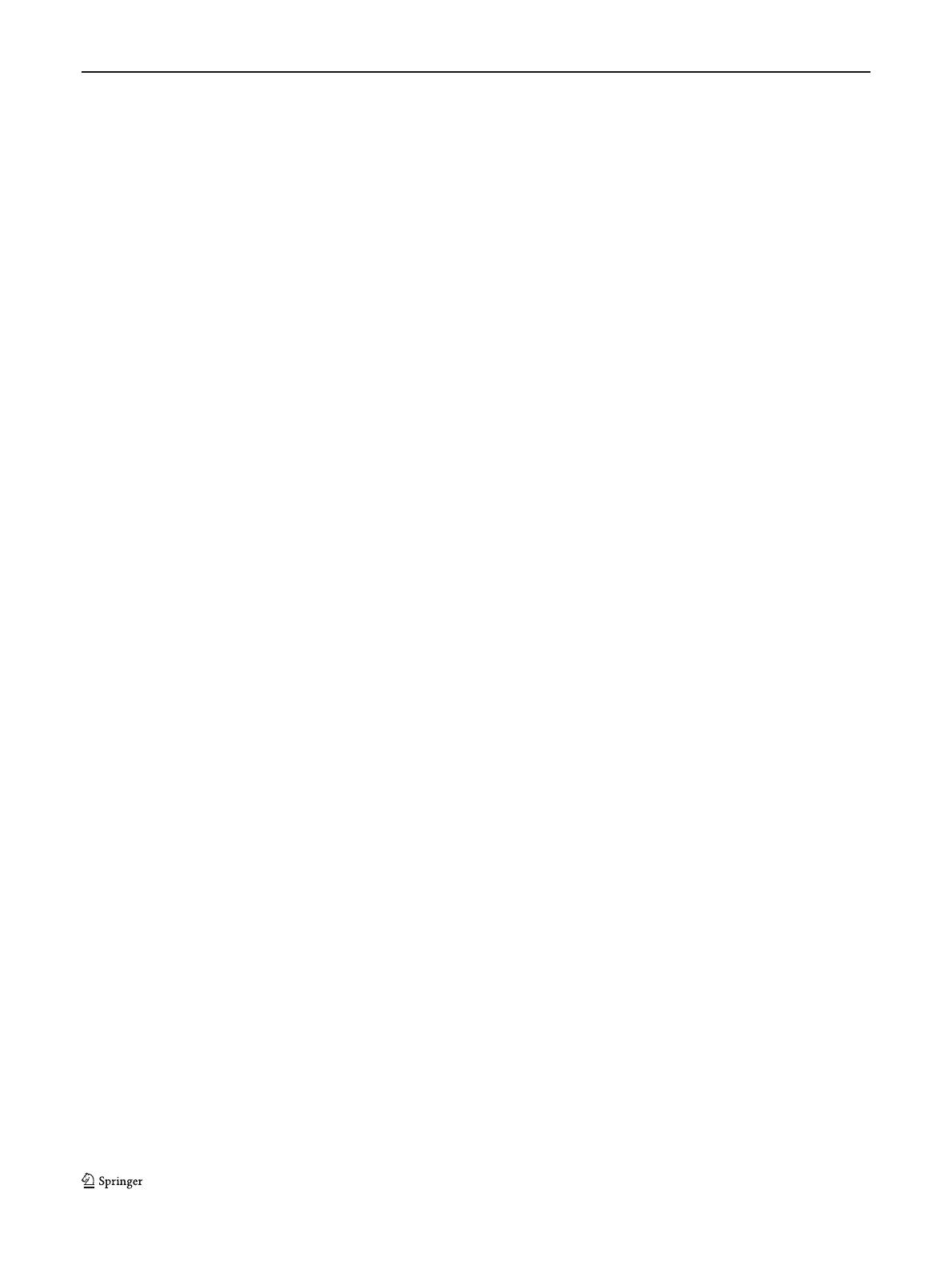
or behaviour^. Chronic, low-level exposure to EDCs in both
humans and animals has been linked to a number of reproduc-
tive disorders (Kookana et al. 2007).
EDCs encompass a wide variety of chemical classes, in-
cluding natural and synthetic hormones, plant constituents,
pesticides, certain compounds in plastics, personal care prod-
ucts, pharmaceuticals, pollutants and by-products of industry
(Damstra et al. 2002). EDCs are widely dispersed in the envi-
ronment and have been found in rivers, estuaries, coastal sam-
ples and even in the arctic (Braga et al. 2005a;Jenssen2006).
Some of the most potent EDCs include steroid estrogens such
as estrone (E1), 17β-estradiol (E2), estriol (E3) and 17α-
ethinylestradiol (EE2). These estrogenic EDCs have been
shown to have adverse affects on the reproductive systems
of marine and freshwater animals at less than 5 ng L
−1
(Jobling
et al. 1998,2002a,b,2006; van Aerle et al. 2001; Pelley
2003). Estrone is a metabolite of estradiol and is an important
pregnancy estrogen. Estradiol is a reproductive hormone nat-
urally excreted by female animals including humans and in
much smaller quantities by males. Ethinylestradiol is the main
ingredient in the female contraceptive pill and hormone re-
placement medication (Trussell 2001;Songetal.2009).
A major source of estrogenic EDCs to natural waterways is
wastewater treatment plant effluent and leachate of sewage
sludge either buried in landfill or applied as fertilizer (Jobling
et al. 2006; Koyama et al. 2006; Thorpe et al. 2009;
Karnjanapiboonwong et al. 2011; Marti and Batista 2014).
Concentrations of estrogenic EDCs in wastewater can range
from nanograms per litre (ng L
−1
) to micrograms per litre (μg
L
−1
) (Ying and Kookana 2005). Other potential sources of
estrogenic EDCs in developed and agricultural catchments
include on-site wastewater management systems, effluent
run-off following manure application or run-off of livestock
wastes (Reneau and Degen 1989; Hanselman et al. 2003;
Zhang et al. 2011).
Water contamination by sewage is common in all island
countries of the Pacific region with many streams and ground-
water supplies currently considered unsafe for human con-
sumption without treatment (UNESCAP 2000). In the porous
carbonate sands of many Pacific islands, groundwater is par-
ticularly susceptible to contamination from poorly performing
sanitation systems. Indeed ground and surface water pollution
associated with septic tank and absorption trench disposal
systems is common in many Pacific countries (Dakers and
Evans 2007). Some soil absorption systems which are used
as on-site treatment systems in these countries can operate
successfully for years, whilst others can fail within weeks of
installation (Beal et al. 2005). Studies by
Karnjanapiboonwong (2011) showed that EDCs that were
present in the effluent from a wastewater treatment plant were
able to reach the groundwater after land application of the
effluent. Given the porous nature of the soil in most Pacific
islands, the steadily increasing human population and greater
use of synthetic compounds, such as the contraceptive pill, it
is quite likely that groundwater may contain elevated concen-
trations of estrogenic EDCs. However, it is also possible that
carbonate sands may act to physically, chemically and/or bio-
logically remove estrogenic EDCs from effluent water.
There is some evidence that EDCs can be effectively de-
graded during transport through porous media (Nakada et al.
2007). Biodegradation of EDCs has been shown to be the
most cost-effective way of removing EDCs relative to ad-
vanced removal mechanisms such as activated carbon, mem-
brane filtration, chemical advanced oxidation and ozonation
(Liu et al. 2009).Usingquartzsandfiltrationsystems,Nakada
et al. (2007) showed a 50 % removal rate of estrogenic EDCs.
Carbonate sands may be particularly effective for the treat-
ment of EDCs (Rasheed et al. 2003). They may be an ideal
candidate for incorporation into wastewater treatment systems
in developing Pacific nations due to their ready availability.
However, the behaviour of EDCs in carbonate sands has not
been studied previously. In particular, it is not understood how
the biodegradation of estrogens will be affected by the degree
of saturation within carbonate sands. Other studies have
shown that unsaturated aerobic soils are more likely to de-
grade EDCs relative to saturated anaerobic soils. Permeable
carbonate sands however are unique in that they have ex-
tremely high surface areas capable of sustaining a large mi-
crobial biomass and contain greater adsorption capabilities
and pH buffering relative to quartz sands (Rasheed et al.
2003; Schöttner et al. 2011). The behaviour and degradation
of EDCs in permeable carbonate sands therefore may be en-
hanced relative to other sands and soils. To date, there have
been no studies that have looked at the removal of EDCs in
carbonate sands under saturated or unsaturated conditions.
The aim of this study was to investigate the behaviour of
estrogenic compounds in permeable carbonate sands under
saturated and unsaturated conditions. A broader goal of this
research was to assist in the development of low-cost on-site
wastewater treatment technologies for EDC removal in devel-
oping Pacific countries.
Materials and methods
Equipment and experimental design
In this study, the main objective was to test the reduction of
EDCs within large columns containing permeable carbonate
sands. A secondary objective was to test whether estrogenic
EDC removal would be affected by the addition of other soils/
materials (e.g. basalt soil, coconut fibre) to some of the sand
columns. Basalt sediment and coconut fibre were used as
amendments as these are readily available and commonly
used with carbonate sand in wastewater treatment systems
throughout the Pacific.
Environ Sci Pollut Res