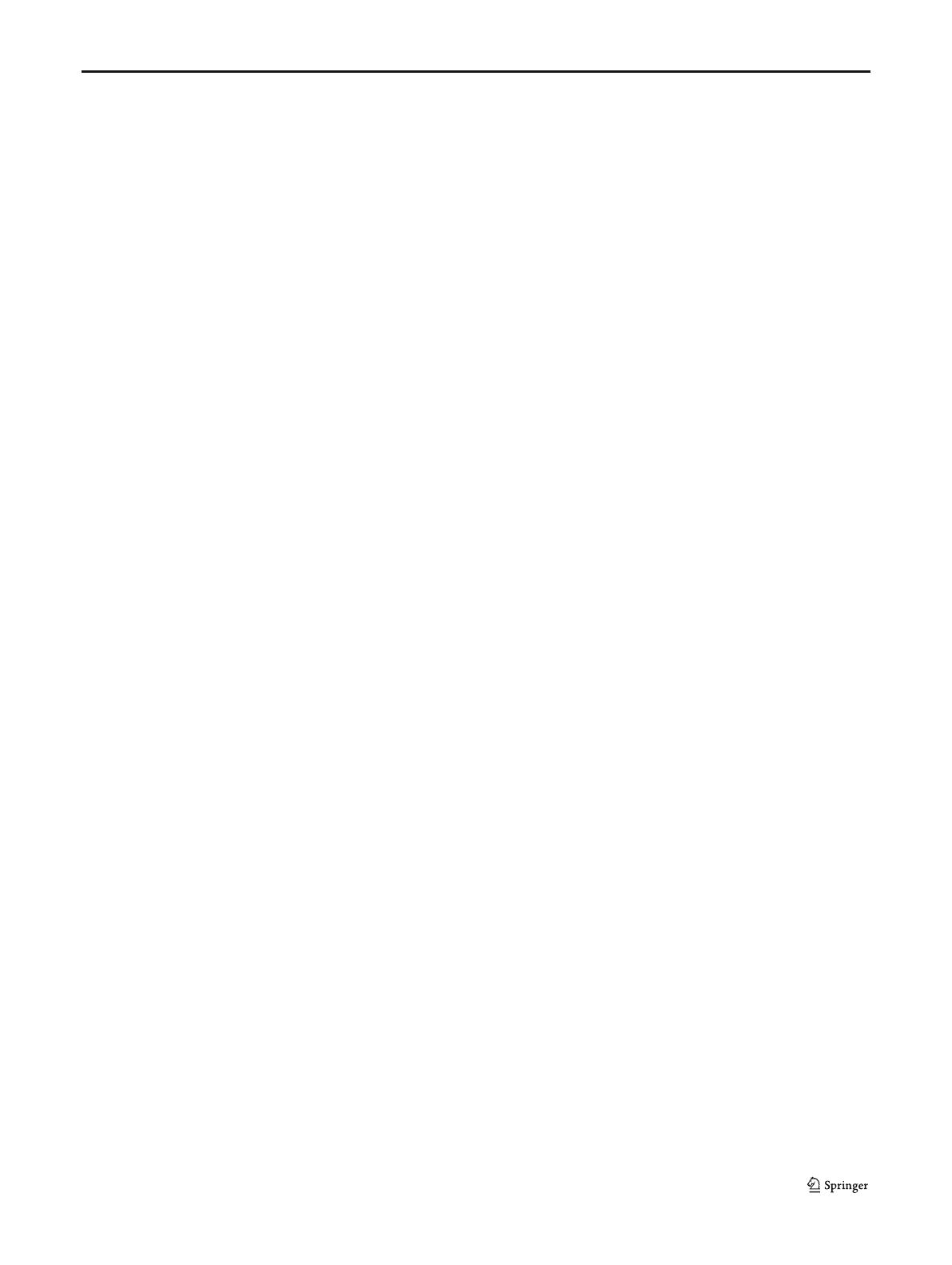
lesions may recapitulate some developmental patterns such as
large band Blaschko lines (Fig. 1f). The long held neural the-
ory based on possible dermatomal distribution is challenged
by many exceptions. The aetiology of the SV pattern remains
overall elusive. Rarely, multiple segmental lesions occur si-
multaneously or not distributed either unilaterally or bilateral-
ly. A clear segmental distribution of the lesions with midline
demarcation, together with the associated features described
in mono-segmental cases (leukotrichia, protracted course),
distinguishes this diagnosis versus vitiligo in bilateral cases.
As in vitiligo, repigmentation may occur, usually under com-
bined therapy (see below), according to a marginal, follicular,
or mixed pattern (Fig. 1g).
Unclassified and Rare Variants
The VGICC classification suggests that focal cutaneous or
mucosal vitiligo (defined as small isolated patch that does
not fit a segmental distribution, and which has not evolved
into vitiligo after a period of at least 2 years) should be until
better consensus on nature and course left within the category
undetermined⁄unclassified vitiligo. Some rare variants are
discussed below.
Vitiligo ponctué/punctata
Lesions present as sharply demarcated depigmented
punctiform 1- to 1.5-mm macules involving any area of the
body (Fig. 1h), and has to be distinguished histopathologically
from guttate hypomelanosis, a common condition with no loss
of melanocytes situated on chronically sun exposed sites such
as the legs and forearms.
Vitiligo Minor/Hypochromic Vitiligo
This disease seems to affect only dark-skinned individuals
(Fig. 1i). ‘Minor’refers to a partial defect in pigmentation.
The relation to true vitiligo comes from pathology and coex-
istence with more typical vitiligo macules. Cutaneous T cell
lymphoma needs to be ruled out by repeated biopsies with
molecular studies of clonality, and this diagnosis cannot be
made without a long-term follow-up [17].
Follicular Vitiligo
This designation refers to a form recently described in a young
black patient of generalized vitiligo that primarily involved
the pigment cell follicular reservoir with limited skin involve-
ment, contrasting with marked generalized hair whitening [18]
(Fig. 1j). As noted later in a small series of patients, contrary to
what occurs in common vitiligo, cutaneous depigmentation
follows hair depigmentation which is situated not only in
vitiliginous areas but also in areas with clinically normal-
appearing skin [19].
Immunopathogenesis of Vitiligo
Intrinsic Abnormalities of Melanocytes and Keratinocytes
Several in vitro and in vivo studies have revealed an altered
redox status, with the presence of oxidative stress in cultured
melanocytes coupled with an increased susceptibility to pro-
oxidant agents [20,21]. Elevated levels of reactive oxygen
species (ROS) have been observed in lesional and non-
lesional skin of vitiligo. The increased ROS production by
melanocytes could result from an external stress, such as ul-
traviolet (UV) radiation exposure or chemical damage
(monobenzone or other phenols). A wide range of metabolic
pathways leads to the uncontrolled generation of ROS, and
several lines of evidence suggest that mitochondria could be
the main source of ROS in vitiligo [22,23]. ROS can also
create a pro-inflammatory environment that will contribute
to the activation of the immune system [24]. Moreover, vitili-
go melanocytes, probably as a consequence of increased in-
tracellular oxidative stress, present abnormalities of signal
transduction pathways compatible with a condition of stress-
induced premature senescence-like phenotype. This is charac-
terized by the production of many proteins among the
senescence-associated secretory phenotypes, including the
production of pro-inflammatory cytokines as interleukin
(IL)-6, matrix metalloproteinase 3 (MMP3), cyclooxygen-
ase-2, insulin-like growth factor-binding protein 3 (IGFB3),
and IGFBP7 [25]. In addition, recent observations showed
that melanocyte exposure to chemical agents (4-TBP—4-ter-
tiary butyl phenol—and MBEH—monobenzyl ether of hy-
droquinone) known to trigger vitiligo induces the disruption
of the folding machinery of the endoplasmic reticulum (ER),
leading to the accumulation of immature proteins and
resulting in the activation of the unfolded protein response
(UPR) [26]. UPR activation results in transient attenuation
of protein synthesis, increased capacity for protein trafficking
through the ER, protein folding transport, and increased pro-
tein degradative pathways, including ER-associated degrada-
tion (ERAD) and autophagy. If these adaptive mechanisms
cannot resolve the protein-folding defect, as in vitiligo, cells
enter apoptosis. The UPR comprises three parallel signalling
branches: PRKR-like ER kinase (PERK)-eukaryotic transla-
tion initiation factor 2α(eiF2α) pathway, activating transcrip-
tion factor 6α(ATF6α), and the inositol-requiring enzyme-1
(IRE1)–X-box-binding protein 1 (XBP1). Genome-wide link-
age analysis followed by a sequencing study in a Chinese
population with vitiligo identified XBP1 as a candidate gene
for vitiligo predisposition, which was then confirmed in a
vitiligo Caucasian cohort [27–29]. More recently, He et al.
Clinic Rev Allerg Immunol (2018) 54:52–67 55