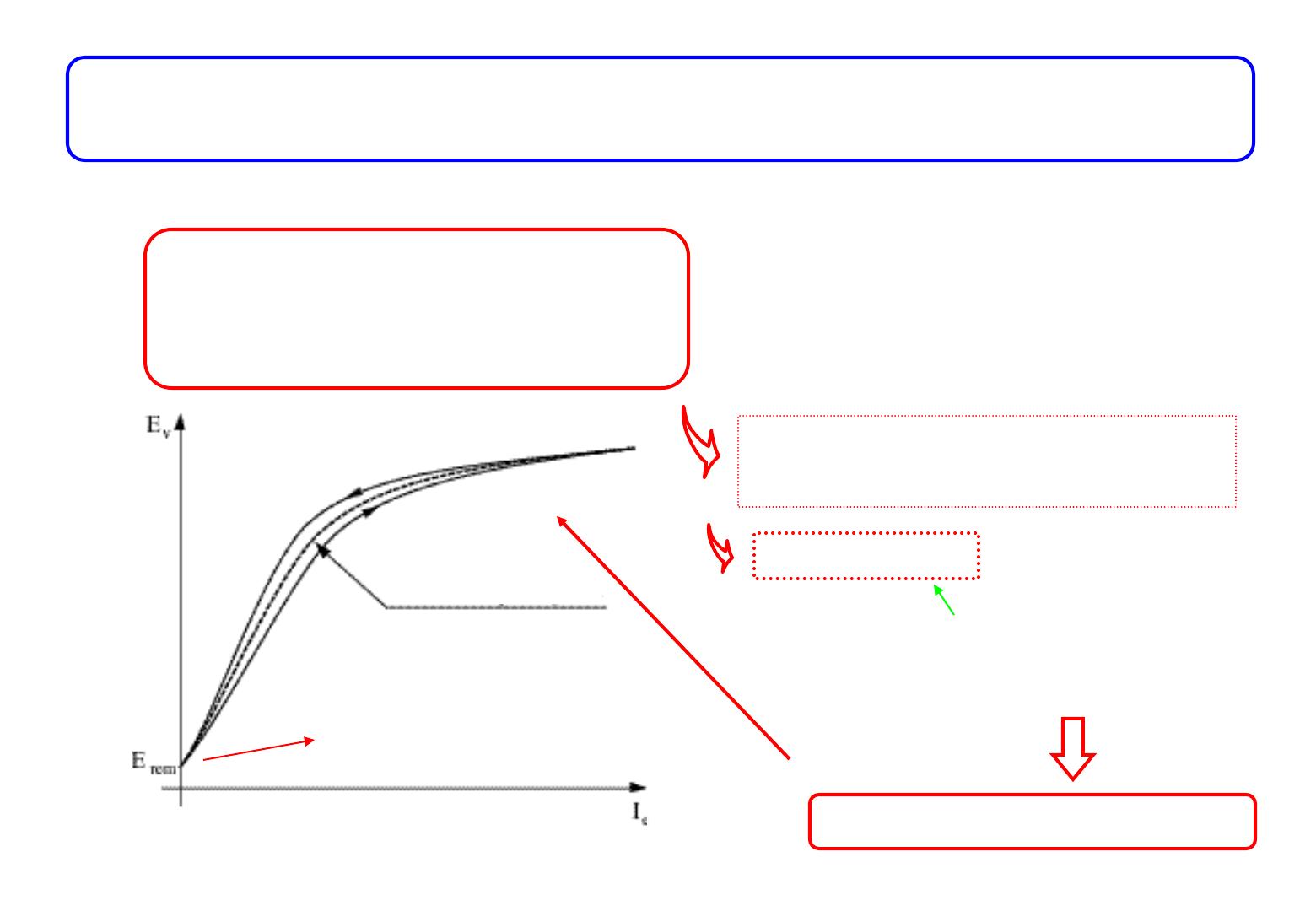
2
No-load characteristic
Evolution of the voltage Evin a stator phase
vs. intensity of the excitation current Ie, for a
given rotation speed and with no generated
stator current
⎩
⎨
⎧
=
=θ
=0I
constantevitesse
avec)I(fE ev
!
Magnetic flux produced
by the inductor and seen by the
stator winding
Non linearity with hysteresis
due due remanent magnetization
•The rotor winding, carrying the DC current Ieand ro ta t in g
at speed ω/p,produces in the airgap asl i ding m.m.f. Fe
(as seen from the stator).
• Fegenerates amagnetic flux density Br(with the sa me
phase) in the airgap, which induces si nu soi da l e.m.f.s Ev
in the stator windings,with aphase lag of
π
/2.
Synchronous machines
average characteristic
average characteristic
with constant speed