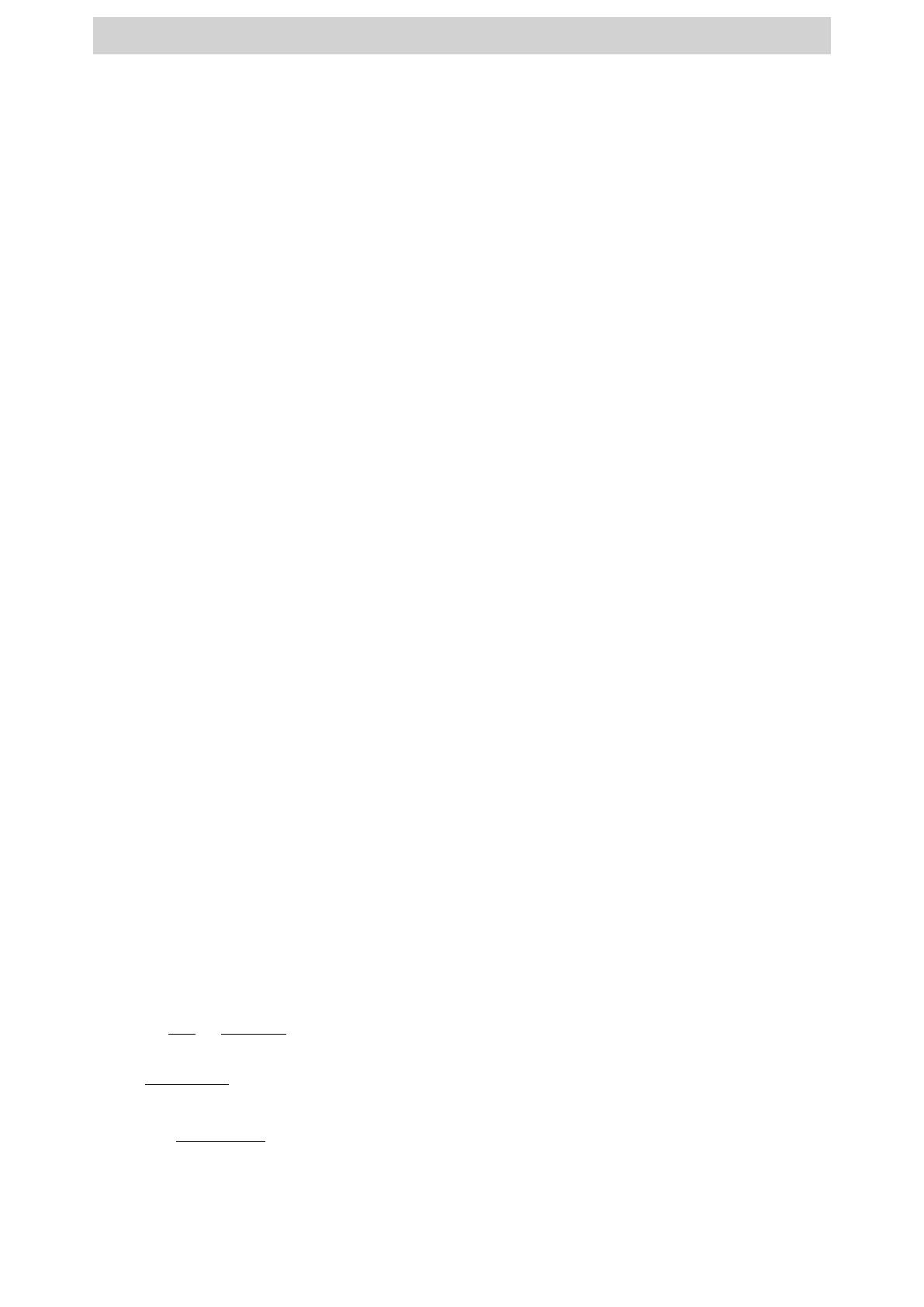
ACCEPTED MANUSCRIPT
4
reaction, the reagents were washed with 10 mL ethyl acetate and a small amount of sodium iodide, then
heated to room temperature (298K) for 9 h and then heated to 338K for 27 h. The resulting solution was
purified by washing, filtering with ethyl acetate and dried in vacuum.
6-(Quinolin-6-ylmethyl) benzene-1,2,3,4,5-pentasulfonic acid (QBPA) was synthesized by reacting the
mixture of intermediate product BQ and concentrated sulfuric acid (molar ratio 1:5) in an ice-water bath for
2 h and then heated to 338K for 4 h. The product was percolated, swashed with ethyl acetate and desiccated
in vacuum.
Fig. S1 shows the FT-IR and 1H NMR spectrums of BQ and QBPA. For BQ: light red, solid, yield =
82%. 1H NMR (400 MHz), δ (ppm) (Fig. 2a): 3.5144 (m, 2H, ArCH2), 7.1918 (t, 2H, ArCH2CCHCH),
7.2924 (d, 2H, ArCH2CCH and NCHCH), 7.8075 (t, 1H, Ar-H), 7.9603 (m, 2H, Ar-H), 8.2243 (d, 2H, Ar-
H), 9.0438 (d, 1H, NCHCHCH-), 9.2104 (d, 1H, NCH-). FT-IR (KBr pellet) (Fig. 2c): 2922.9 and 2852.0
cm-1 (ν C-H, aliphatic fatty chain), 1032.6-1230.6 cm-1 (ν C-N, fatty amines) and 3060.1-3254.2 cm-1 (ν C-H,
aromatic). For QBPA: red, liquid, yield= 63%. 1H NMR (400 MHz), δ (ppm) (Fig. 2b): 3.3532 (m, 5H, SO3H),
3.9652 (m, 2H, ArCH2), 7.1901 (t, 2H, ArCH2CCHCH), 7.2903 (t, 2H, ArCH2CCH and NCHCH) 9.0481
(d, 1H, NCHCHCH-), 9.2019 (d, 1H, NCH-). FT-IR (KBr pellet) (Fig. 2d): 1355.2 and 1192.8 cm-1 (νR-SO2-
OH), 1022.8 cm-1 (ν C-N, fatty amines), 2920.6 and 2850.5 cm-1 (ν C-H, aliphatic fatty chain), 2976.6-3260.9
cm-1 (ν C-H, aromatic).
2.2. Materials and reagents for inhibition tests
The size of mild steel was 3 cm ×2 cm ×1 cm for weight loss measurements and 0.5 cm ×0.5 cm ×0.5
cm for surface analysis while the exposed area of electrochemical experiments was 1 cm2. Before the tests,
all specimens were carefully polished by a sequence of emery papers of grade Nos. 400, 800, 1200 and 2000,
and then rinsed with distilled water, degreased with absolute ethyl alcohol and finally dried at room
temperature.
Analytical grade HCl (37.5%, purchased in Chongqing Chuandong Chemical Co., LTD) was diluted
with distilled water to acquire the test solution.
2.3. Weight loss experiments
In this study, weight loss experiments were taken according to the standard methods [11]. The corrosion
parameters (corrosion rate CRW, the surface coverage θ, corrosion inhibition efficiency ηW) were calculated
using the following equations [12, 13]:
(1)
(2)
(3)
ACCEPTED MANUSCRIPT