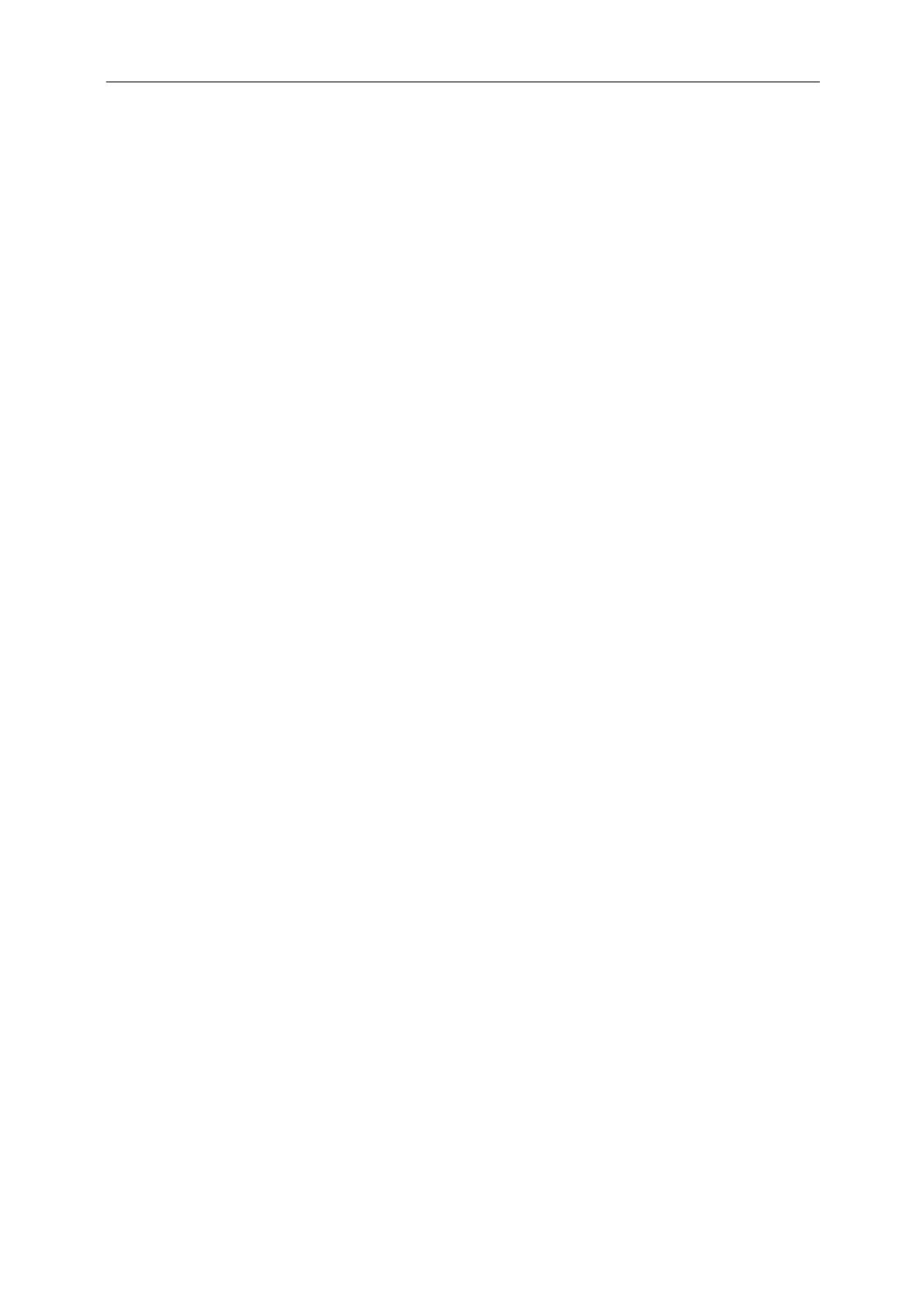
Table of Contents
Table of Contents
A. Abstracts
A.1 Zusammenfassung 1
A.2 Summary 3
B. Abbreviations 5
1. Introduction 8
1.1 The Epstein-Barr virus 8
1.1.1 Classification and structure 8
1.1.2 Epidemiology and clinical manifestations 9
1.1.3 Latent and lytic infection 11
1.1.4 EBV infection in the natural host 12
1.1.5 EBV infection in vitro 14
1.2 Immune responses to EBV 15
1.2.1 Humoral immune response 15
1.2.2 The CD8+ T cell response to EBV 16
1.2.3 The CD4+ T cell response to EBV 18
1.3 EBV in the immunocompromised 21
1.4 Immunological approaches to control EBV infection 22
1.4.1 T cell therapy of EBV-associated lymphomas 23
1.5 Aim of the work 24
2. Materials and Methods 25
2.1 Laboratory equipments 25
2.2 Cell biology materials and methods 26
2.2.1 Eukaryotic cell culture materials 26
2.2.2 General conditions for eukaryotic cell culture 27
2.2.3 Separation of peripheral blood mononuclear cells from whole blood 28
2.2.4 Preparation of pooled human sera for T cell media 29
2.2.5 Establishment and culture of lymphoblastoid cell lines 29
2.2.6 Generation and maintenance of T cell lines and clones 30
2.2.7 Preparation of dendritic cells from PBMCs 31
2.2.8 Methods for T cell phenotype and function analysis 32
2.2.8.1 Flow cytometric analysis of T cells 32