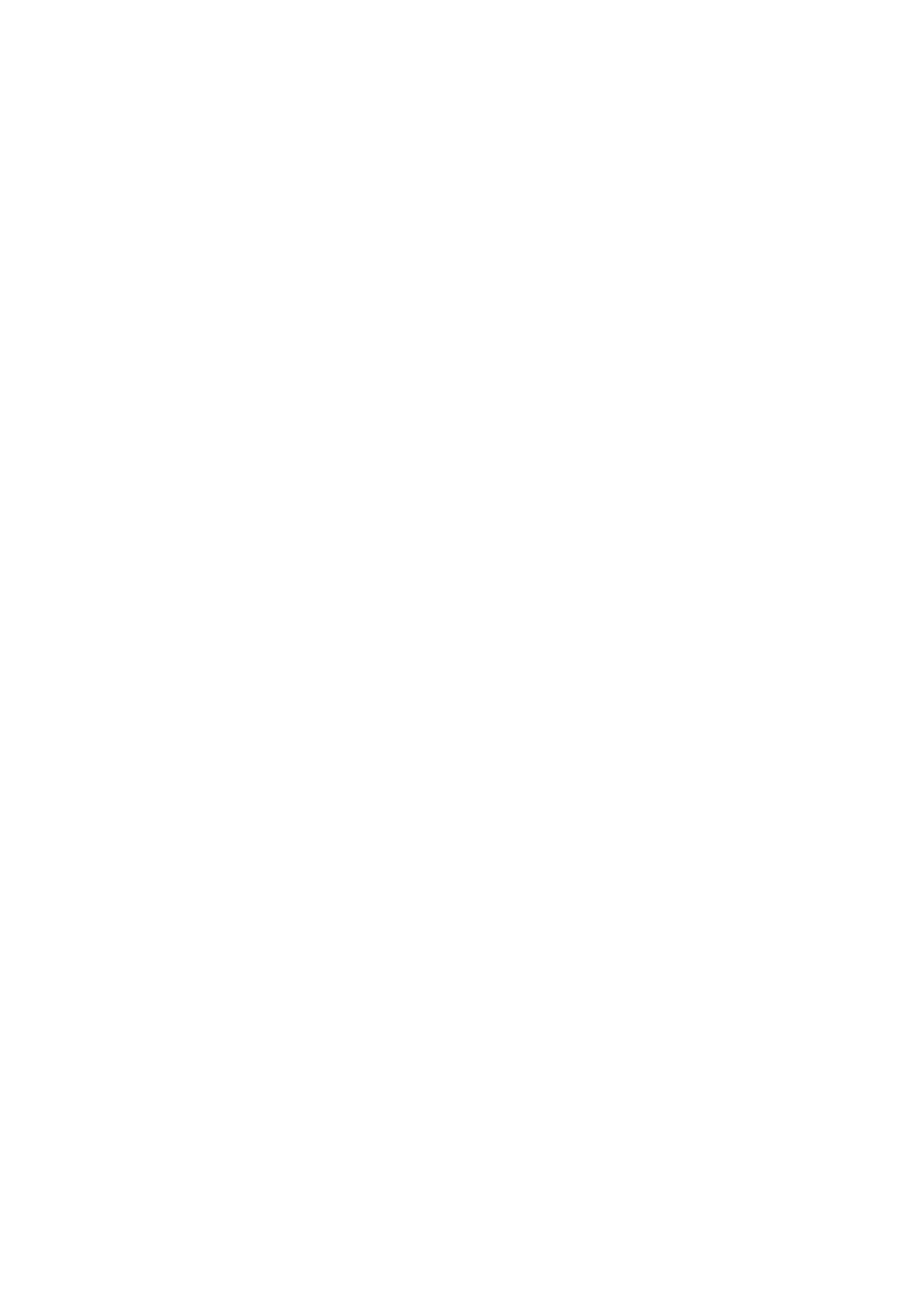
3
AGRADECIMENTOS
Agradeço ao meu orientador, prof. João Antonio Pêgas Henriques, por ter me
recebido em seu laboratório em 2009 como aluna de iniciação científica e ter
acreditado em meu potencial até hoje. Obrigada de coração.
Ao meu coorientador, prof. Guido Lenz, por toda a atenção e pelas ótimas
ideias. É uma honra tê-lo como colaborador do meu trabalho.
Ao Programa de Pós-Graduação em Biologia Celular e Molecular e às
agências de fomento, CAPES e FAPERGS, pelas oportunidades.
Ao Luciano e à Sílvia, por serem tão atenciosos e prestativos.
Aos membros da banca examinadora pela colaboração.
À Diana, por toda a paciência e pelas puxadas de orelha quando necessário.
Muito obrigada por ter acreditado na minha capacidade e por todo o tempo
dedicado a me ensinar. Este trabalho não teria sido feito sem a colaboração da
Diana.
Às minhas queridas amigas Larissa, Bruna e Victória, pela companhia e pelas
risadas. O ambiente de trabalho é muito mais agradável com a presença de
vocês.
Às colombianas lindas, Grethel e Lyda. Aos meninos, Cristiano, André, Joel, e
Iuri. Às meninas que não estão mais no lab 210, mas que moram no meu
coração, Clara, Patrícia, Juliane, Ana, Renata e Fernanda. Às meninas do
Genotox, especialmente Miriana, Rose, Márcia e Nusha. À Aline, uma amiga para
todas as horas. Muito obrigada a todos vocês pelo carinho e amizade.
Aos meus pais, Marli e Genézio, e minha irmã, Luciane. Muito obrigada por
depositarem tanto amor e confiança em mim. Amo vocês.
Ao meu namorado/marido Paulo. Muito obrigada por ser o cara mais carinhoso
e paciente do universo. Sem o seu apoio nada disso teria acontecido. Amo-te,
meu nerd preferido.
Muito obrigada a todos!