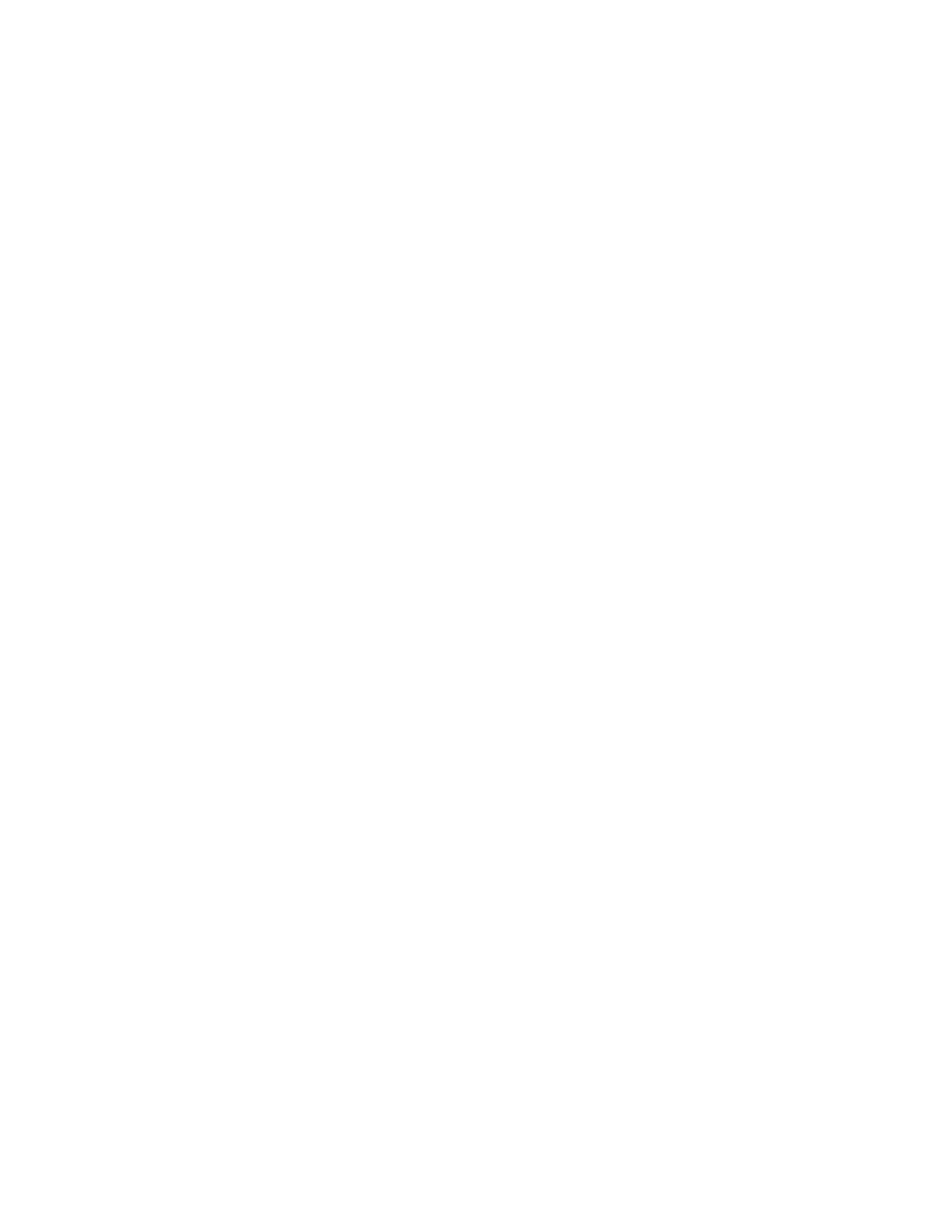
iv
Acknowledgements
This thesis would not have been possible without the guidance and support of several
individuals who have contributed in one form or another. First and foremost, I would like
to thank my supervisor Dr. Frank Jirik. His vast repository of knowledge and endless
assistance and direction was a prominent reason for my success.
I would also like to acknowledge all of my lab members, as they all contributed to this
work in one way or another. Everyone’s contribution was invaluable.
Dr. Helen Buie helped with much of the microCT work, and was an extreme asset to the
completion and success of this work.
I would also like to acknowledge Leona Barclay’s vast contribution in the sectioning and
staining of the majority of histology slides.
My committee members, Dr. Shirin Bonni and Dr. Steven Boyd also provided a
tremendous amount of influence and supportive guidance through the learning process of
this research.
Lastly, I would like to acknowledge the tremendous influence my family and friends
provided throughout. They all taught me to strive for nothing but perfection. I would
especially like to thank my parents and Laura Evans for their indispensable support that
motivated my passionate ambition for success.