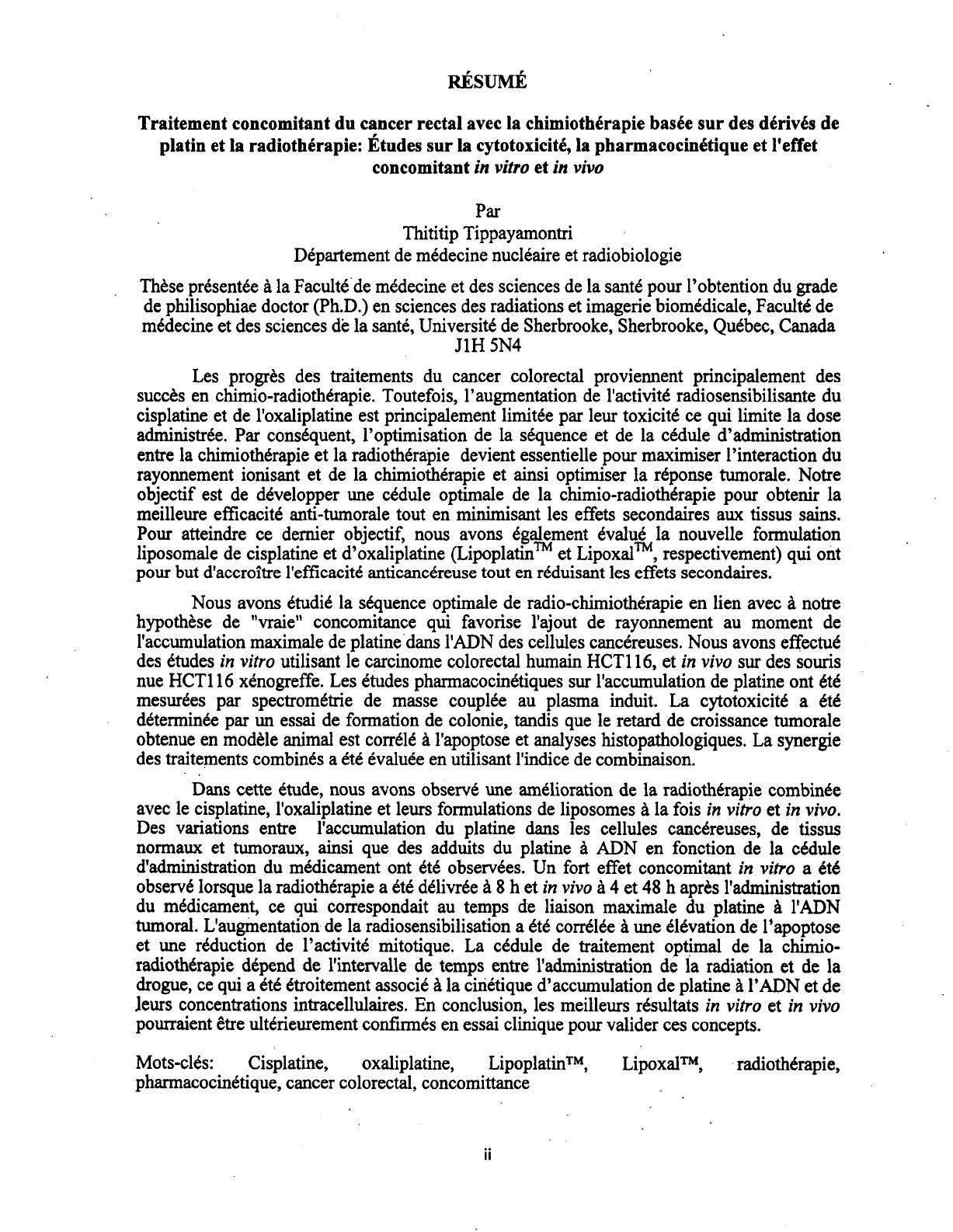
RESUME
Traitem ent concomitant du cancer rectal avec la chimiotherapie basee sur des derives de
platin et la radiotherapie: Etudes sur la cytotoxicity, la pharmacocinetique et l'effet
concomitant in vitro et in vivo
Par
Thititip Tippayamontri
Departement de medecine nucleaire et radiobiologie
Thyse pr6sentye a la Faculty de mddecine et des sciences de la sante pour l’obtention du grade
de philisophiae doctor (Ph.D.) en sciences des radiations et imagerie biom&licale, Faculty de
mydecine et des sciences de la santy, University de Sherbrooke, Sherbrooke, Quybec, Canada
J1H 5N4
Les progrys des traitements du cancer colorectal proviennent principalement des
succys en chimio-radiothyrapie. Toutefois, 1’augmentation de l'activity radiosensibilisante du
cisplatine et de l'oxaliplatine est principalement limitye par leur toxicity ce qui limite la dose
administrye. Par consequent, l’optimisation de la syquence et de la cydule d’administration
entre la chimiothyrapie et la radiothyrapie devient essentielle pour maximiser l’interaction du
rayonnement ionisant et de la chimiothyrapie et ainsi optimiser la ryponse tumorale. Notre
objectif est de dyvelopper une cydule optimale de la chimio-radiothyrapie pour obtenir la
meilleure efficacity anti-tumorale tout en minimisant les effets secondaires aux tissus sains.
Pour atteindre ce dernier objectif, nous avons ygalement yvalud la nouvelle formulation
liposomale de cisplatine et d’oxaliplatine (Lipoplatin™ et Lipoxal™, respectivement) qui ont
pour but d'accroitre l'efficacity anticancyreuse tout en ryduisant les effets secondaires.
Nous avons ytudiy la syquence optimale de radio-chimiothyrapie en lien avec a notre
hypothyse de "vraie" concomitance qui favorise l'ajout de rayonnement au moment de
1'accumulation maximale de platine dans l'ADN des cellules cancyreuses. Notts avons effectuy
des ytudes in vitro utilisant le carcinome colorectal humain HCT116, et in vivo sur des souris
nue HCT116 xenogreffe. Les ytudes pharmacocinytiques sur 1'accumulation de platine ont yty
mesuryes par spectromytrie de masse couplee au plasma induit. La cytotoxicity a yty
dyterminye par un essai de formation de colonie, tandis que le retard de croissance tumorale
obtenue en modyie animal est corryie a l'apoptose et analyses histopathologiques. La synergie
des traitements combinys a yty evaluye en utilisant l'indice de combinaison.
Dans cette ytude, nous avons observy une amyiioration de la radiothyrapie combinye
avec le cisplatine, l'oxaliplatine et leurs formulations de liposomes a la fois in vitro et in vivo.
Des variations entre 1'accumulation du platine dans les cellules cancyreuses, de tissus
normaux et tumoraux, ainsi que des adduits du platine k ADN en fonction de la cydule
d'administration du mydicament ont yty observyes. Un fort effet concomitant in vitro a yty
observy lorsque la radiothyrapie a yty dyiivrye k 8 h et in vivo a 4 et 48 h apr£s l'administration
du mydicament, ce qui correspondait au temps de liaison maximale du platine k l'ADN
tumoral. L'augmentation de la radiosensibilisation a yty corryiye a une yiyvation de l’apoptose
et une ryduction de l’activity mitotique. La cydule de traitement optimal de la chimio-
radiothyrapie dypend de l'intervalle de temps entre l'administration de la radiation et de la
drogue, ce qui a yty ytroitement associy k la cinytique d’accumulation de platine k l’ADN et de
Jeurs concentrations intracellulaires. En conclusion, les meilleurs rysultats in vitro et in vivo
pourraient etre ultyrieurement confirmys en essai clinique pour valider ces concepts.
Mots-ciys: Cisplatine, oxaliplatine, Lipoplatin™, Lipoxal™, radiothyrapie,
pharmacocinytique, cancer colorectal, concomittance