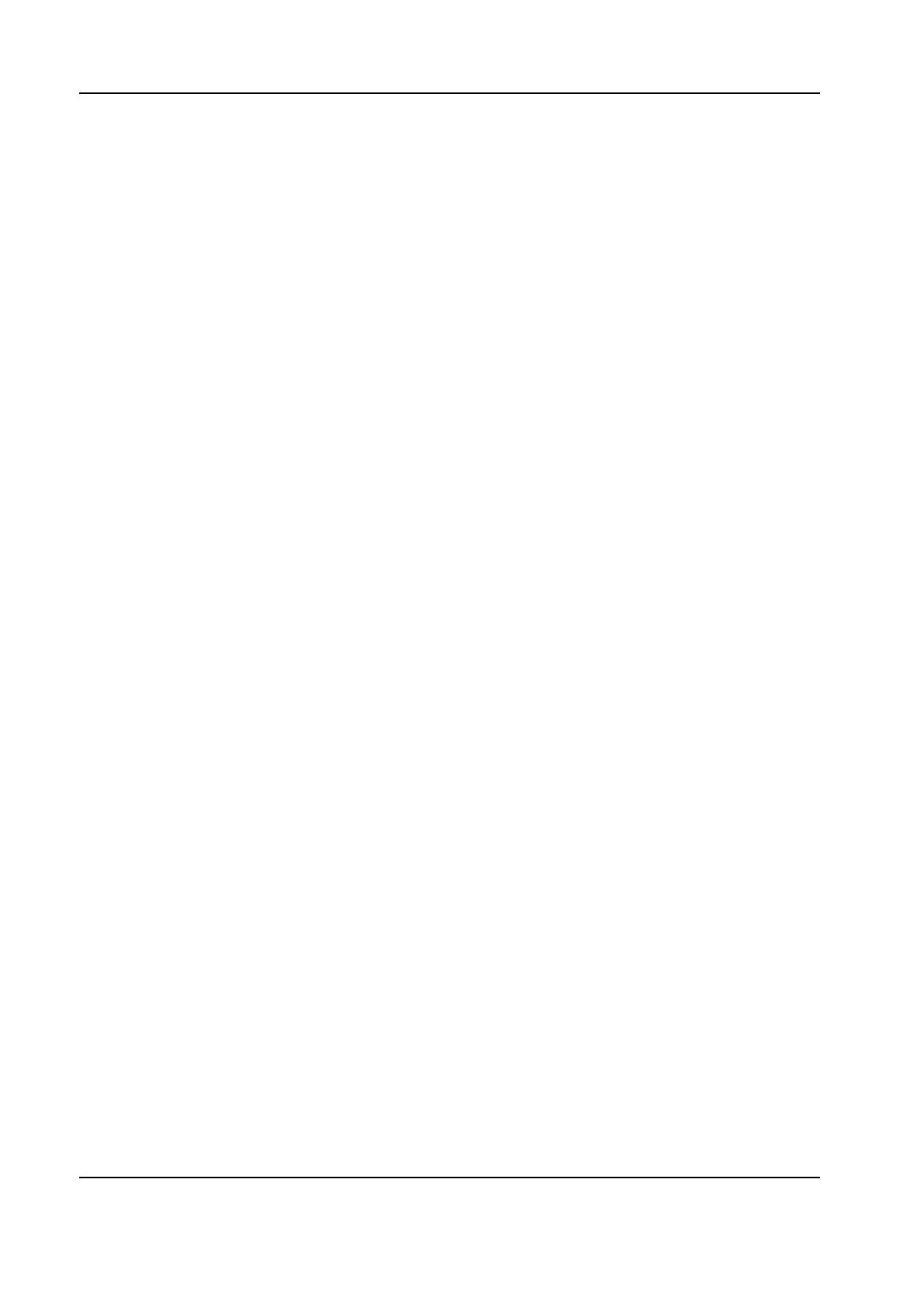
Discussió
158
prometedorsdinslaviroteràpiadelcàncer.Amés,adiferènciademoltsaltresvirus,existeixun
coneixementraonabledelasevabiologiaipermetenlaincorporaciódegensexògensquepoden
atorgarpropietatsúniquesalsvirus(CodyandDouglas,2009).Algunsadenovirusoncolítics
genèticamentmodificats,principalmentOnyx‐015,jahanestattestatsenassajosclínics,incloent
unampliventalldetipustumoralsidiferentsrutesd’administració.Lesdadesclíniques
obtingudesindiquenunbonperfildeseguretatitoxicitat,exceptuantalgunsefectesadversos
preocupantsdesprésdel’administraciód’elevadesdosis(Nemunaitisetal.,2001;Aghiand
Martuza,2005).Siensfixemenl’eficàcia,lamajoriaderespostesobservadesvanresultar
transitòries,ieltractamentnovasercapaçd’alterarsignificativamentl’evoluciódelamalaltia
(Habibetal.,2002;Hamidetal.,2003;Smalletal.,2006).Així,aquestsresultatsindicavenla
necessitatd’unamillorasubstancialdelsadenovirusoncolíticsperpoderproduirrespostes
efectivesenhumans.
Peraltractamentefectiudetumorsenestatavançat,esrequereixl’administració
sistèmicadel’agentoncolíticpertald’arribaratotselsfocustumoralsimetàstasis.Amés,
l’administraciósistèmicapermetunadistribuciómésuniformedelviruspeltumor,ajudanta
l’eradicaciód’aquest(Weinetal.,2003).Noobstant,elvirusestrobaambdiverseslimitacions
desprésdeseradministratsistèmicament:primerament,elviruséseliminatràpidamentdel
torrentsanguinipelfetge(Alemanyetal.,2000)oinactivatperlaunióacèl∙lulessanguínies,a
anticossosneutralitzantsoalcomplement(Paratoetal.,2005).Així,nomésunapetitaproporció
delvirusadministratarribaaltumor;uncopaltumor,unadelescaracterístiquesdelsadenovirus
quelimitenméslasevaeficiènciaéslaincapacitatdedistribuir‐seeficientmentpertoteltumor.
Algunesdelesestratègiesempradesenlabibliografiaconsisteixenenmutarcertsgensvirals,tals
comE1B‐19Kolaproteïnai‐leader(Yanetal.,2003;Liuetal.,2004;Subramanianetal.,2006),o
ensobreexpressarlaproteïnaADP(Tothetal.,2004)permillorarlasortidadelvirusdelacèl∙lula,
encaraqueaquestesmodificacionss’hanassociatambgreusefectesadversoscomladisminució
delaproduccióviralolapèrduadelesfuncionsimmunomoduladoresd’E3(Pilderetal.,1984;
Wangetal.,2003).Noobstant,unadeleslimitacionsmésimportantsaladispersiódelvirusésla
matriudeltumor,queimposabarreresfísiquesqueelvirusdifícilmentpottravessar(Kimetal.,
2006).Unaestratègiaques’hamostratmolteficientpersuperaraquestesbarreresconsisteixen
aportaralviruseinesperdestruir‐les,armant‐losambtransgenstalscomproteasespertrencarla
matriu(Kimetal.,2006)oenzimsconvertidorsdeprodroguesambefectebystanderperles
barreresfibròtiques(Chenetal.,2004).Malgratelsbeneficisqueaportaaquestaestratègia,la
capacitatd’encapsidaciódelvirusestàlimitadaal105%delamidadelgenomasalvatge(Bettet