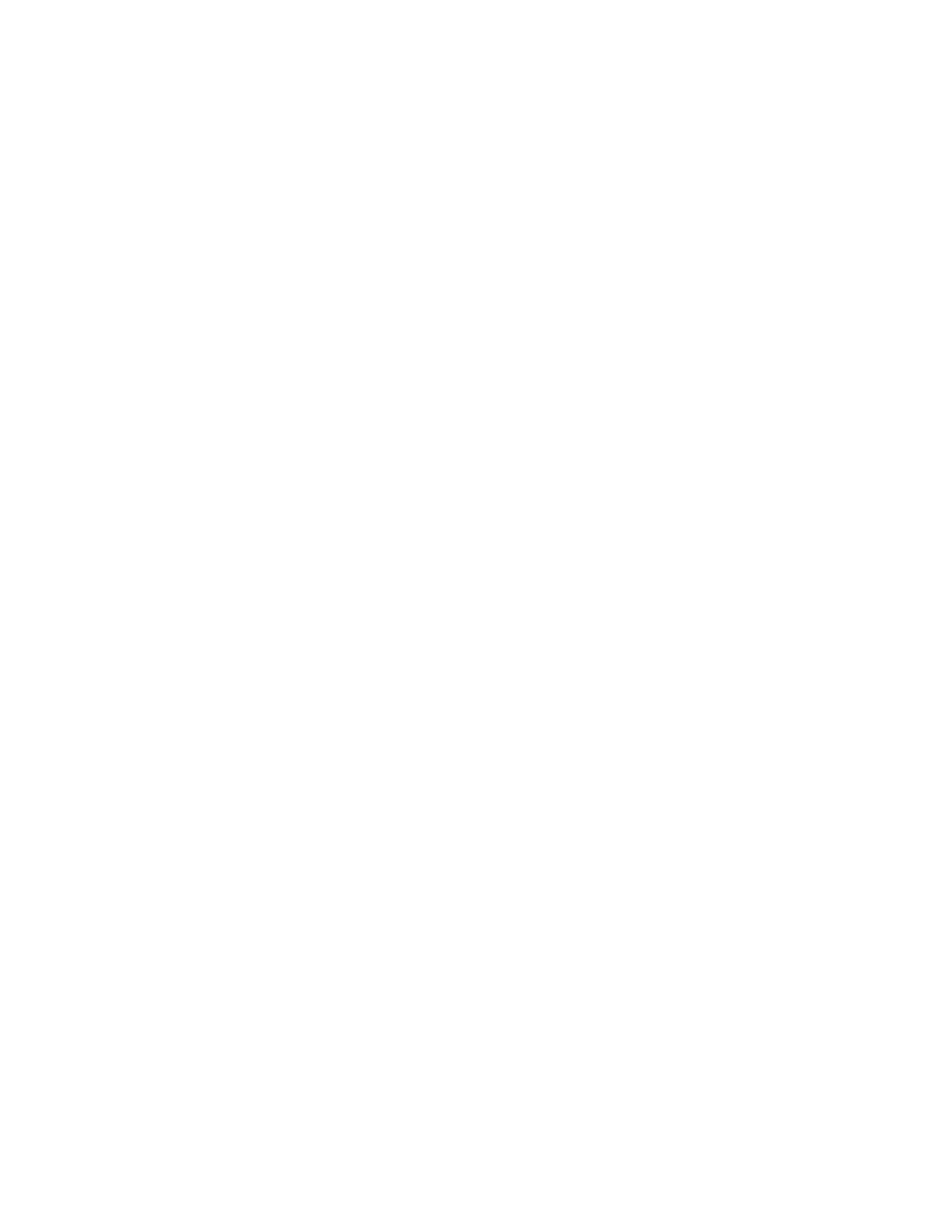
ii
Résumé long
Les bifidobactéries sont des bactéries candidates potentielles aux probiotiques à
cause de leurs effets bénéfiques sur la santé de l’homme incluant la prévention et/ou
traitement des gastro-entérites, intolérance au lactose, cancer, hypercholestérolémie, etc.
Une des propriétés intéressantes des bifidobactéries est leur capacité à moduler certaines
fonctions du système immunitaire et remédier à certaines pathologies immunologiques.
Cependant, les études rapportées démontrant les effets immunomodulateurs des
probiotiques sont basées sur des modèles utilisant des cellules bactériennes vivantes et
limités à certaines souches probiotiques. L’effet des composants issus de la lyse cellulaire
dans le tractus gastro-intestinal sur le système immunitaire demeure mal connu, d’où alors
la nécessité d’élucider les mécanismes d’immunostimulation induits par ces derniers. Le
but de ce projet est d’étudier le potentiel immunomodulateur de certaines souches de
bifidobactérie isolées d’humains et utilisées à l’état non viable et d’explorer les mécanismes
moléculaires impliqués dans cette action immunomodulatrice. Notre étude visait d’une part,
à évaluer les effets de certains composants cellulaires de bifidobactérie notamment le
contenu cytoplasmique brut, la paroi cellulaire, et les exopolysaccharides (EPS) sur la
réponse immunitaire, et d’autre part, caractériser à l’échelle moléculaire les composants à
pouvoir immunomodulateur et exploiter leur propriété pour produire des anticorps
monoclonaux permettant de détecter spécifiquement les bifidobactéries dans les aliments.
Trois souches locales de bifidobactéries, B. thermoacidophilum RBL81, RBL82 et RBL64
(fèces de bébés) productrices d’EPS ont été étudiées et comparées au Bifidobacterium lactis
Bb12 (souche de référence). Des souches ATCC de bifidobactéries ont également été
utilisées pour développer des anticorps monoclonaux.
Les résultats obtenus montrent des effets immunostimulants dépendant de la
souche/espèce, du composant cellulaire et de la dose utilisée. Une meilleure stimulation de
la prolifération cellulaire (Indice de stimulation =16) est obtenue avec la paroi cellulaire de
B. lactis Bb12 (40 µg/ml). Le contenu cytoplasmique stimule la réponse immunitaire à un
niveau moindre que la paroi, par contre, les EPS bruts ou fractionnés n’induisent aucun
effet. La stimulation de la prolifération à été confirmée par le dosage des cytokines révélant