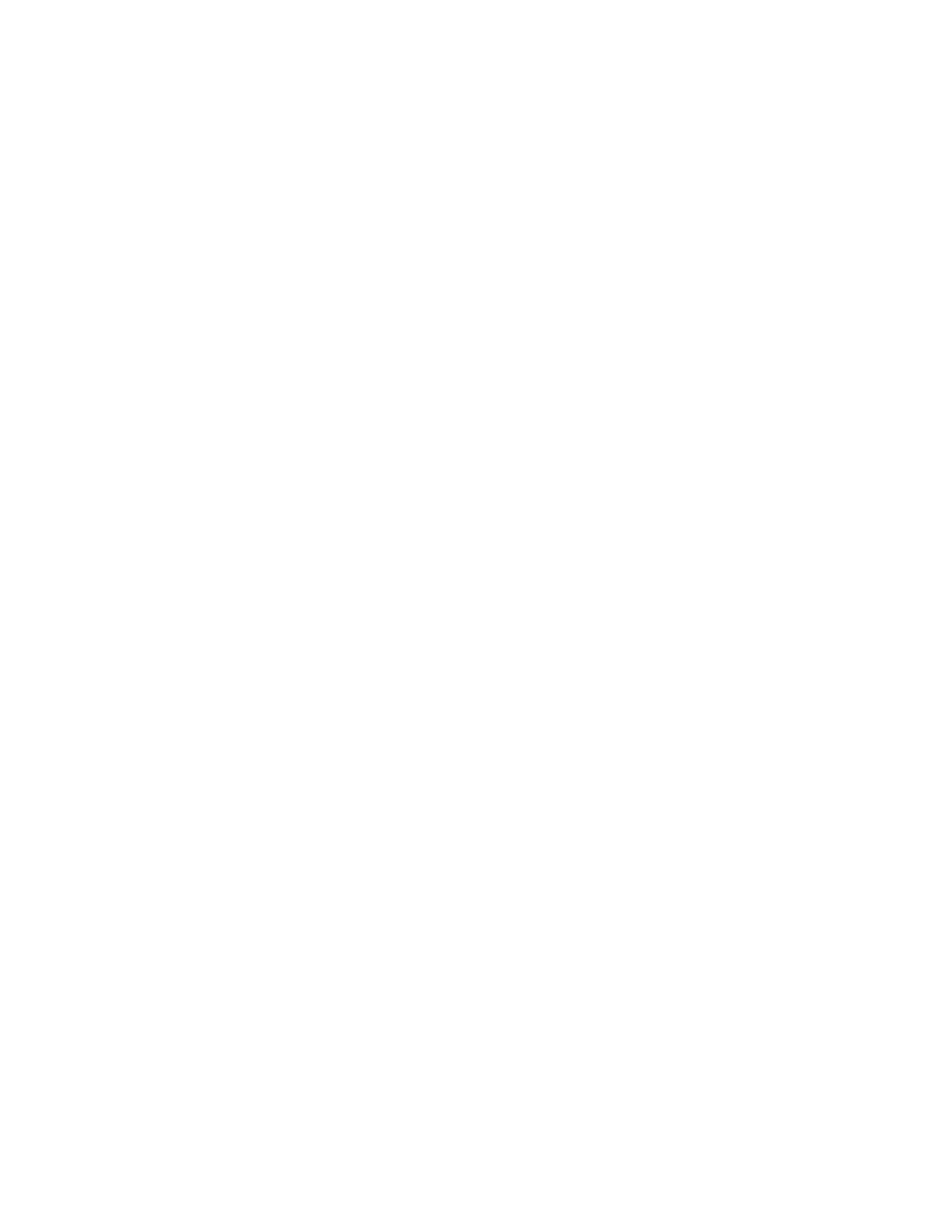
iii
Résumé
Les lymphocytes Th17 sont une sous-population de lymphocyte T découverte récemment et
dont la particularité est de pr -17. Ces cellules ont été impliquées dans le
développement de maladies auto-immunes. Toutefois, les mécanismes régulant les
fonctions des lymphocytes Th17 dans ces maladies ne sont pas très bien connus. Plusieurs
études indiquent que les intégrines liant le collagène sont des molécules de costimulation
pour les lymphocytes T effecteurs. De plus, ces intégrines ont été impliquées dans le
développement de plusieurs maladies auto-immunes. On les retrouve entre autres sur les
lymphocytes T
intégrines liant le collagène chez les lymphocytes Th17 sont inconnues. Nous avons montré
Th17,
Nous avons également observé que les collagènes de
type I et de type II costimulent -1 - - dans les
lymphocytes Th17 humains activés par le récepteur des cellules T (TCR) alors que le
collagène de type IV, liant
cytokines. Nous avons également montré que les lymphocytes T expriment un autre
récepteur pour le collagène, soit DDR1. Nos résultats suggèrent que ce récepteur
adhésion, mais plutôt dans la migration des lymphocytes T dans le
collagène tridimensionnel. Nous avons trouvé que les lymphocytes Th17 expriment DDR1,
mais que celui-Nos résultats
ont mis en évidence le rôle des voies de signalisation MAPkinases ERK, JNK et la voie
PI3K/AKT -17 suite à la stimulation par le TCR
-17 par
transcription RORc, qui joue un rôle central dans la différenciation Th17
-17expression de RORc nécessite
les voies MAPkinase ERK et PI3K/AKT qui sont aussi augmentées par le collagène. Nos
résultats indiquent que participe à
ce fait peut réguler à la hausse le développement des maladies auto-immunes dans les tissus
riches en collagène.