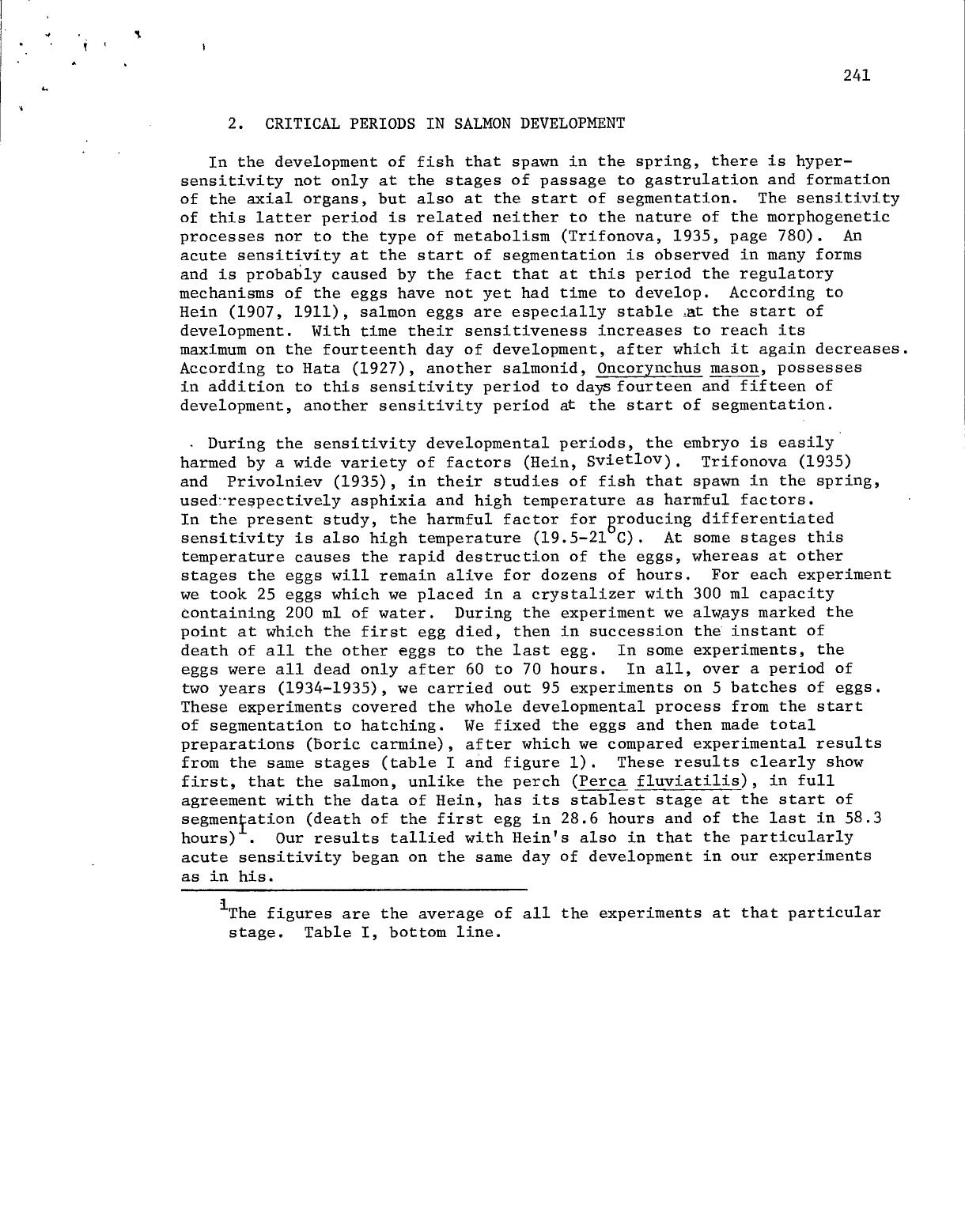
241
2.
CRITICAL PERIODS IN SALMON
DEVELOPMENT
In the
development
of
fish that spawn
in the spring,
there is hyper-
sensitivity not only at
the stages of passage to gastrulation and formation
of the axial
organs,
but
also at
the
start
of segmentation. The
sensitivity
of
this
latter
period is related neither to
the nature of the
morphogenetic
processes nor to
the type of
metabolism
(Trifonova, 1935,
page
780).
An
acute sensitivity at
the
start
of segmentation
is observed
in
many forms
and
is probably caused by
the
fact that at this period
the
regulatory
mechanisms
of the
eggs
have
not yet had time to develop. According to
Hein
(1907, 1911),
salmon eggs
are
especially
stable
at
the start
of
development. With time their sensitiveness increases to reach its
maximum on the
fourteenth day
of
development, after which it again decreases.
According to
Hata (1927),
another
salmonid,
Oncorynchus mason, possesses
in addition
to this sensitivity period to
daysfourteen and
fifteen
of
development, another sensitivity period at
the
start
of segmentation.
During
the
sensitivity developmental periods,
the
embryo is easily
harmed by
a
wide variety
of factors (Hein,
Svietlov)
. Trifonova (1935)
and
Privolniev (1935),
in
their studies
of
fish that spawn
in the spring,
used
-
respectively
asphixia
and
high temperature
as
harmful
factors.
In the present study,
the
harmful
factor for
groducing
differentiated
sensitivity is also high temperature
(19.5-21 C).
At
some
stages
this
temperature
causes the
rapid
destruction of the eggs, whereas at other
stages the
eggs will remain alive
for
dozens
of
hours.
For
each experiment
we took
25
eggs which we placed
in a crystalizer
with
300 ml
capacity
containing
200 ml
of water.
During
the
experiment we always marked
the
point
at which
the
first egg died, then in succession the instant of
death
of
all
the
other eggs to
the
last egg. In
some experiments,
the
eggs were all dead only after
60
to
70
hours. In all, over
a
period
of
two years
(1934-1935),
we carried
out
95
experiments
on
5
batches
of
eggs.
These experiments covered
the
whole developmental process from
the
start
of segmentation
to hatching. We fixed
the
eggs
and
then
made total
preparations (boric carmine), after which we compared experimental results
from
the
same
stages (table
I
and figure
1).
These results clearly
show
first, that
the salmon,
unlike
the
perch (Perca fluviatilis),
in full
agreement
with
the data of Hein,
has its stablest
stage
at
the
start
of
segmentation (death
of the first egg
in
28.6
hours
and of the
last
in
58.3
hours)
.
Our
results tallied with Hein's also
in
that
the
particularly
acute sensitivity began
on the
same day
of
development
in
our experiments
as in his.
1
The
figures are the average of
all
the
experiments at that particular
stage. Table I,
bottom
line.