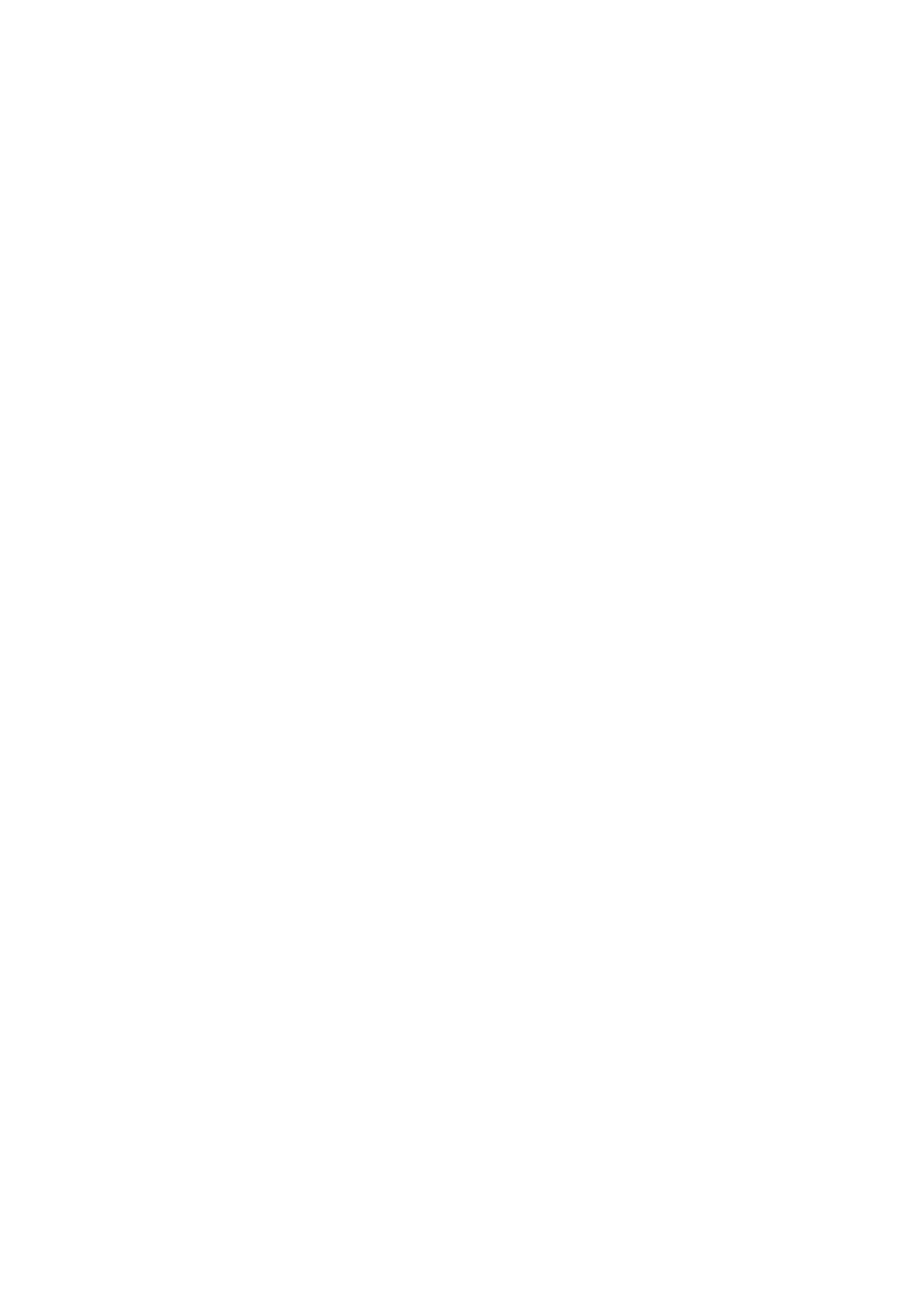
UNIVERSITECLAUDEBERNARDͲ LYON1
Présidentdel’Université
ViceͲprésidentduConseild’Administration
ViceͲprésidentduConseildesEtudesetdelaVie
Universitaire
ViceͲprésidentduConseilScientifique
SecrétaireGénéral
M.FrançoisͲNoëlGILLY
M.leProfesseurHamdaBENHADID
M.leProfesseurPhilippeLALLE
M.leProfesseurGermainGILLET
M.AlainHELLEU
COMPOSANTESSANTE
FacultédeMédecineLyonEst–ClaudeBernard
FacultédeMédecineetdeMaïeutiqueLyonSud–
CharlesMérieux
UFRd’Odontologie
InstitutdesSciencesPharmaceutiquesetBiologiques
InstitutdesSciencesetTechniquesdelaRéadaptation
DépartementdeformationetCentredeRechercheen
BiologieHumaine
Directeur:M.leProfesseurJ.ETIENNE
Administrateurprovisoire:M.leProfesseur
G.KIRKORIAN
Directeur:M.leProfesseurD.BOURGEOIS
Directeur:MmelaProfesseureC.
VINCIGUERRA.
Directeur:M.leProfesseurY.MATILLON
Directeur:M.leProfesseurP.FARGE
COMPOSANTESETDEPARTEMENTSDESCIENCESETTECHNOLOGI
FacultédesSciencesetTechnologies
DépartementBiologie
DépartementChimieBiochimie
DépartementGEP
DépartementInformatique
DépartementMathématiques
DépartementMécanique
DépartementPhysique
DépartementSciencesdelaTerre
UFRSciencesetTechniquesdesActivitésPhysiqueset
Sportives
ObservatoiredeLyon
PolytechLyon
EcoleSupérieuredeChimiePhysiqueElectronique
InstitutUniversitairedeTechnologiedeLyon1
InstitutUniversitairedeFormationdesMaîtres
InstitutdeScienceFinancièreetd'Assurances
Directeur:M.leProfesseurF.DeMARCHI
Directeur:M.leProfesseurF.FLEURY
Directeur:MmeleProfesseurH.PARROT
Directeur:M.N.SIAUVE
Directeur:M.leProfesseurS.AKKOUCHE
Directeur:M.leProfesseurA.GOLDMAN
Directeur:M.leProfesseurH.BENHADID
Directeur:MmeS.FLECK
Directeur:MmelaProfesseureI.DANIEL
Directeur:M.C.COLLIGNON
Directeur:M.B.GUIDERDONI
Directeur:M.P.FOURNIER
Directeur:M.G.PIGNAULT
Directeur:M.C.VITON
Directeur:M.R.BERNARD
Directeur:MmelaProfesseureV.MAUMEͲ
DESCHAMPS