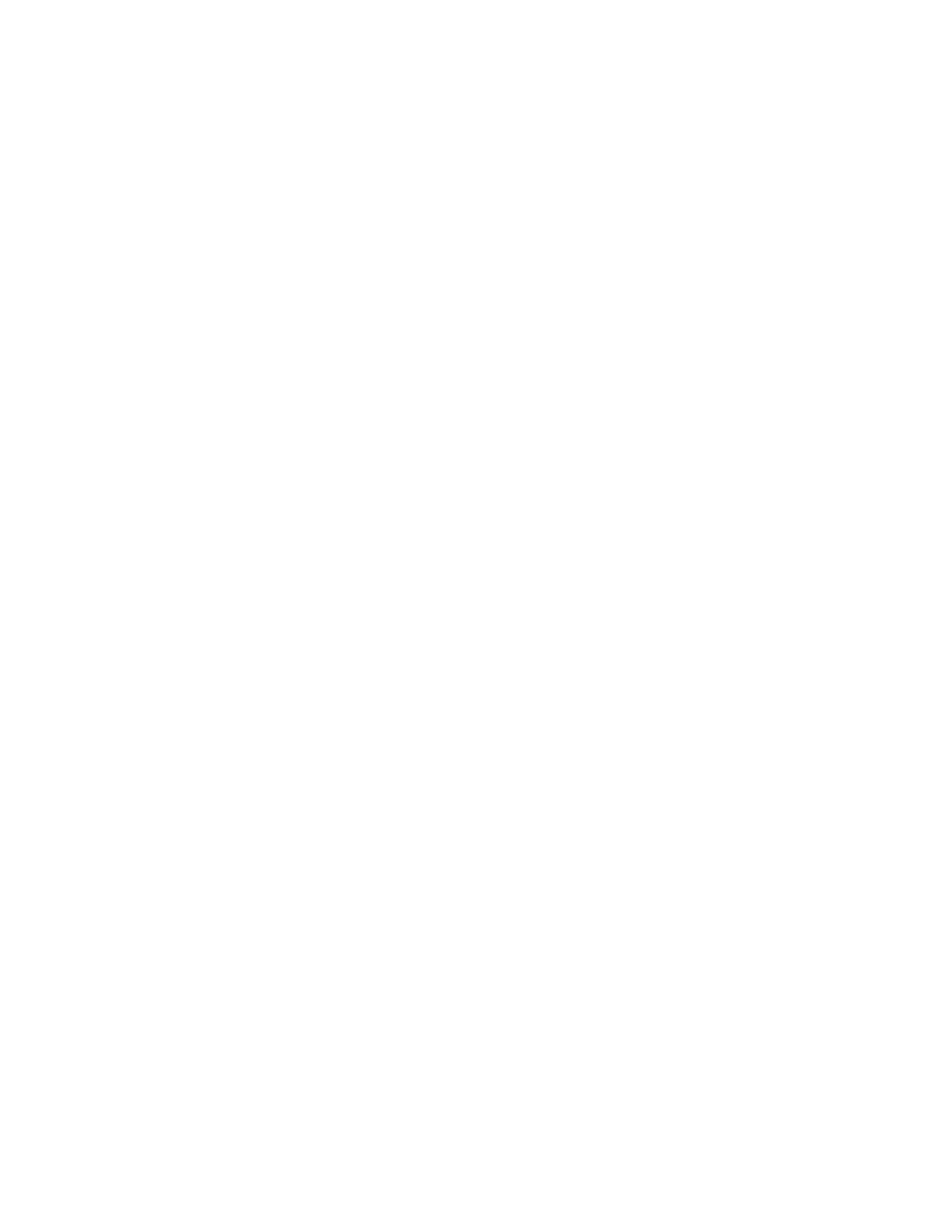
Remerciements
Je tiens d’abord à remercier mon directeur de recherche, John Mackay pour m’avoir
donné la chance et l’opportunité d’entreprendre un projet de maîtrise dans son laboratoire.
Ce projet m’as permis de développer mes connaissances et mon expertise scientifique et de
m’épanouir dans un environnement de travail agréable et enrichissant.
Je veux aussi remercier les professionnels de recherche du laboratoire Isabelle,
Brian, Sébastien et Claude qui ont grandement contribué à mon apprentissage en répondant
patiemment à mes questions et en me transmettant avec plaisir leur bagage de
connaissances ainsi que leur grande expérience de travail.
J’exprime ma reconnaissance envers Armand Séguin et son équipe au Service
canadien des forêts qui ont grandement participé à la production des résultats contenus dans
ce mémoire. Merci particulièrement à Isabelle Duval, qui m’a généreusement accueillie
dans son environnement de travail et a eu la patience de m’enseigner de nouvelles
techniques.
Je remercie aussi mon compagnon de bureau et de salle informatique, Guillaume,
avec qui j’ai eu beaucoup de plaisir à travailler et à discuter. Ta présence a été essentielle au
maintien de ma santé mentale et m’as permis de décompresser lorsque nécessaire.
Je ne veux surtout pas oublier mon ami Alexandre et mon compagnon de vie,
Mikael, tous deux de grand amoureux des sciences, qui ont toujours été présents pour aller
boire un café et discuter de nos projets. Vous m’avez permis de me défouler lorsque les
résultats étaient décevants soit, tout le monde le sait, les trois quarts du temps et m’avez
aidée à trouver de nouvelles solutions qui se sont parfois avérées fructueuses.
Finalement, il ne faut surtout pas oublier mes parents pour leur soutien et leur
amour. Vous êtes mes plus grands admirateurs et je vous en suis reconnaissante.