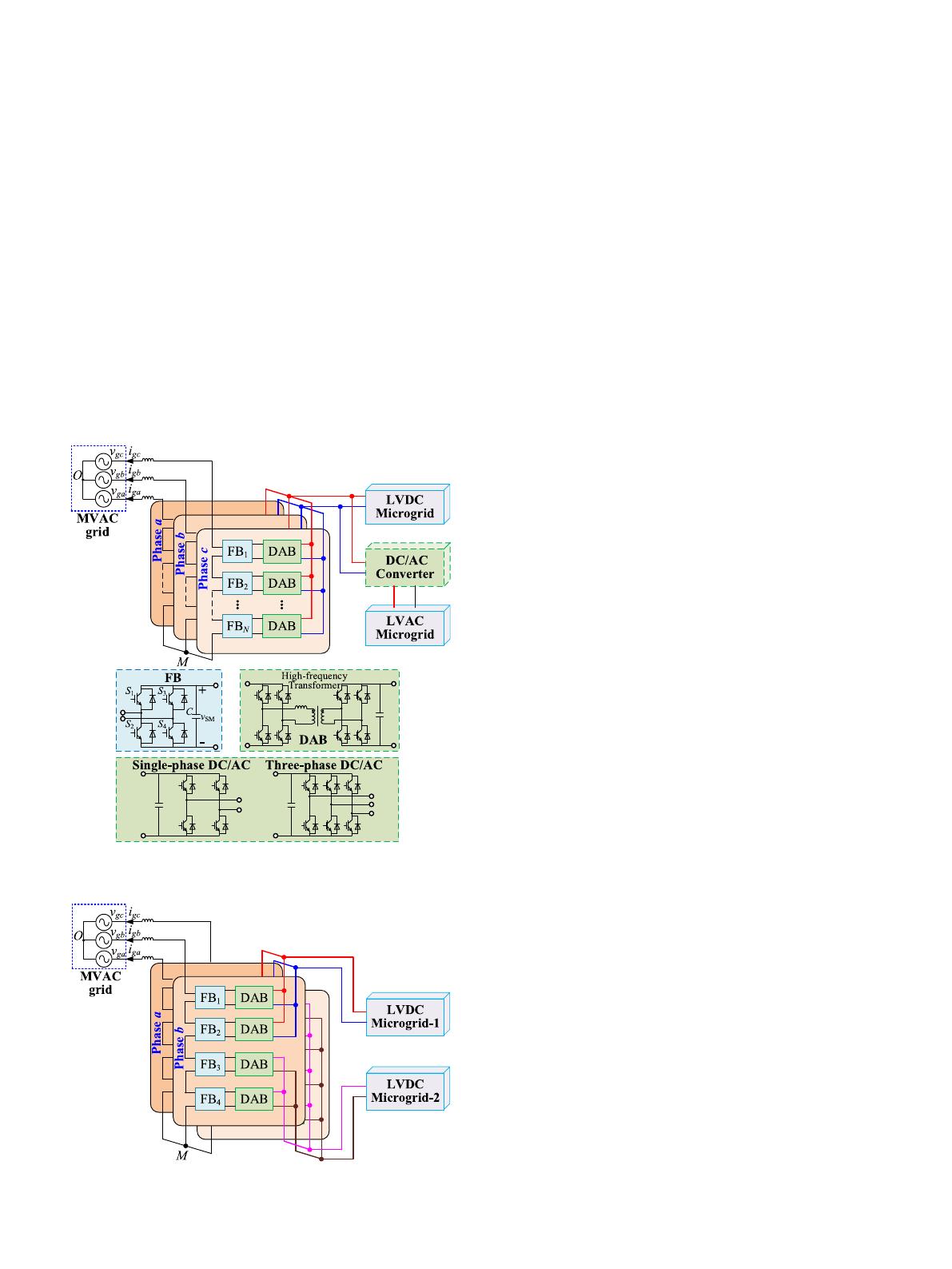
Applied Energy 306 (2022) 118140
2
1. Introduction
1.1. Backgrounds and motivation
For the past two decades, the proportion of electricity in global en-
ergy consumption increase from 17% in 2000 to 22% in 2018 [1]. With
the rapid growth of the economy and deterioration of the environment,
gas emission reduction and carbon neutrality are becoming important
global recognition [2]. The electricity, especially that generated by clean
energy, is gaining much more popularity, of which the electric hybrid
vehicles is a good example. It is estimated that by 2050, the growth rate
of electricity consumption will reach 80% to 90% [3], and it will rapidly
increase the demand for renewable clean energy [4].
The microgrid is an effective solution for the integration and con-
sumption of renewable energy. Conventionally, the AC microgrids are
applied in low-voltage applications, where all distributed generations
(DGs) and loads are connected to the common AC bus, such as smart
buildings, military areas, and rural sectors [5,6]. However, in recent
years, the DC microgrid has been drawing much attention. On the one
hand, it is more economical to integrate renewable energy resources
(such as wind power and PV) and energy storage systems (ESSs) into DC
systems rather than AC systems [7]. On the other hand, a vast demand
for DC power is raised by modern loads like variable-speed drives for
elevators [8]. In addition, electric vehicles (PEVs) also appear as a
crucial modern load in future DC distribution systems, and extensive
novel electronic loads (such as high-quality and highly-efcient DC
lighting systems) have already been integrated into modern houses. The
increasing DC sources and DC loads provide a strong motivation to shift
the mainstream AC microgrids to DC or hybrid microgrids. However, the
widespread AC power system promotes the hybrid microgrids concept as
the preferable candidate, considering its compatibility [9].
In general, the hybrid microgrids are mainly designed for low-
voltage applications, where the AC terminal is connected to the low-
voltage AC (LVAC) microgrids, and the DC terminal is connected to
the low-voltage DC (LVDC) microgrids [10,11]. The AC terminal and DC
terminal are interconnected by bidirectional AC/DC power converters,
and the AC and DC DGs and loads can be connected to the corresponding
terminal. However, to connect the medium-voltage AC (MVAC) grid, the
bulky and volume-occupying line-frequency transformers are usually
necessary.
With the development of remote area mine sites [12] and DC electric
ships [13], the medium-voltage DC (MVDC) microgrid is gaining more
attention in various applications [14]. Therefore, multiple intercon-
nection schemes have been proposed to realize power conversion be-
tween the MVDC and the LVDC system [15]. In addition, with the
increase of electrical power consumption, the efciency of the power
interchange can be greatly improved by the integration of the MVAC
grid. Therefore, it is necessary to realize the direct power integration
between the MVAC grid and MVDC microgrids through multilevel
converter technology [16]. Considering the DC microgrids can better
consume the renewable DGs, and the AC appliance still accounts for the
major part of power loads, it is necessary to connect the MVDC terminal
to the MVAC terminal in the hybrid microgrids together with LVDC and
LVAC microgrids.
1.2. Existing hybrid microgrids
Conventionally, the grid frequency transformer is applied to realize
the interconnection between the medium-voltage and low-voltage sys-
tems [17]. These transformers are usually bulky and heavy, occupying a
large volume. In addition, the high-power grid-frequency transformer
can be expensive [18]. Therefore, to realize exible power conversion
and meet the above interfacing requirements of the multi-terminal
hybrid microgrids, multiple mainstream structures have been pro-
posed in the past decades. One of the popular topologies is motivated by
the cascaded H-bridge (CHB) converter-based power electronic trans-
former (PET) [19]. This topology realizes power conversion between
MVAC and LVDC terminals [20], as shown in Fig. 1. It uses a CHB
converter as the main structure, where the AC output terminal of the
CHB converter is connected to the MVAC grid. The dual active bridge
(DAB) converters are connected to each full-bridge (FB) submodule (SM)
in the CHB converter, and the output terminals of DABs are connected in
parallel to increase the power rating of the LVDC microgrid. The hybrid
microgrids connect the MVAC grid with the LVDC microgrid without a
grid frequency transformer. Moreover, to increase the exibility of this
topology, a DC/AC converter is usually included to provide an addi-
tional terminal for the LVAC microgrid. It is noted that the LVAC grids
can be integrated into the single-phase and three-phase AC grids.
Therefore, the single-phase and three-phase DC/AC converters are
applied individually for different AC grids. In this interconnection
scheme, the power difference between LVDC and LVAC microgrids will
not be inuenced by the power rating of the CHB converter, and the two
microgrids controllers can be designed independently. However, it lacks
the interconnection exibility for more microgrids of different voltage
levels. The improved three-terminal hybrid AC/DC microgrids are pro-
posed in Fig. 2 [21]. As shown in the gure, the DAB converters are
Fig. 1. The CHB-based hybrid AC/DC microgrids in [20].
Fig. 2. The CHB-based multi-terminal hybrid AC/DC microgrids in [21].
Q. Xiao et al.