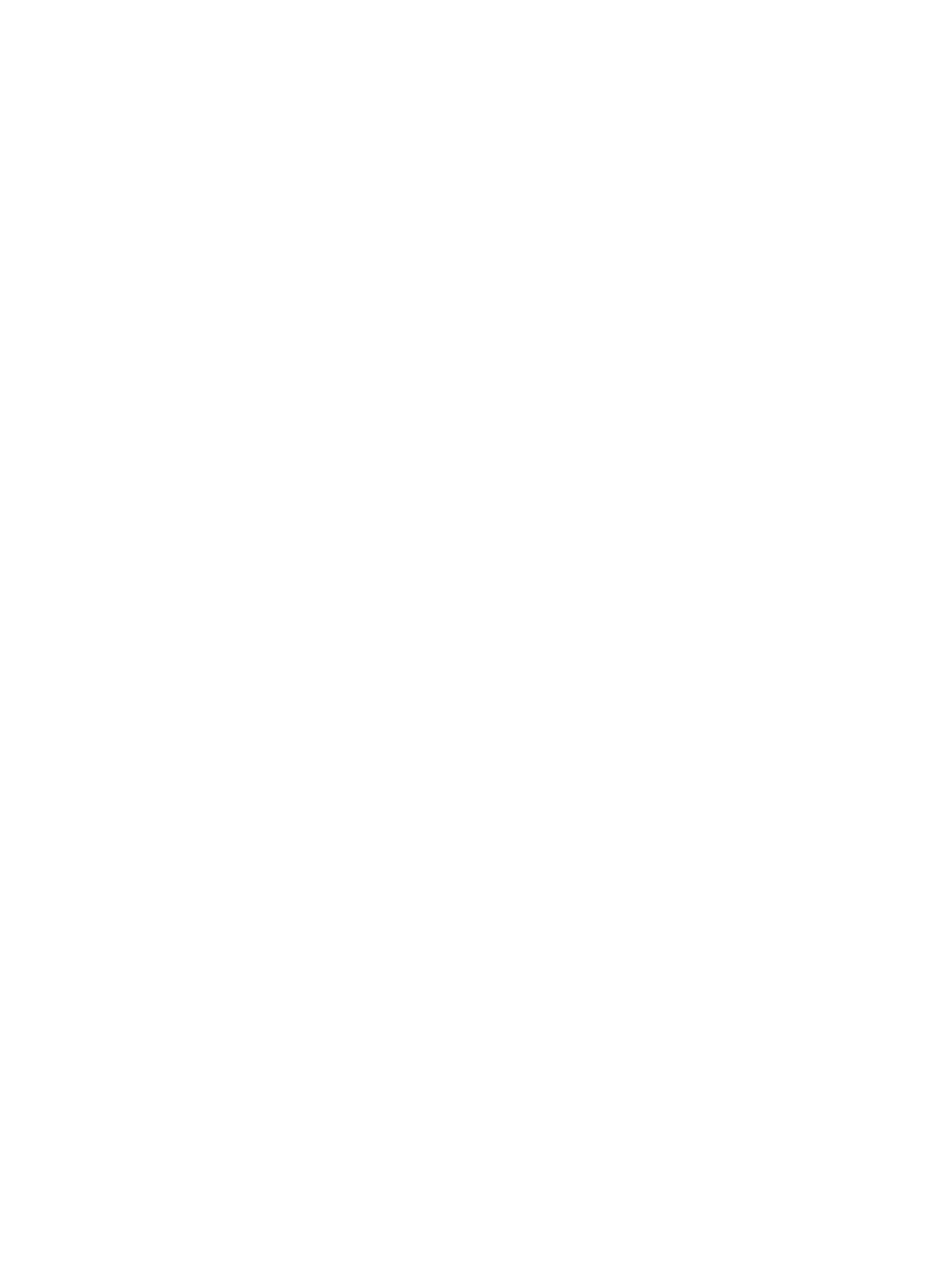
2V.E. Fortov et al. / Physics Reports 421 (2005) 1–103
3.1.1. Motion of a particle in the central field ........................................................................ 9
3.1.2. Orbit motion limited (OML) approximation .................................................................... 12
3.1.3. Accuracy of the OML approximation ......................................................................... 14
3.1.4. Charging in a weakly collisional regime ....................................................................... 15
3.1.5. Charging in a strongly collisional regime ...................................................................... 18
3.1.6. Transitions between the charging regimes ...................................................................... 19
3.2. Charging in anisotropic plasmas .................................................................................... 19
3.3. Other effects important for particle charging .......................................................................... 23
3.4. Role of microparticles in plasma charge balance ...................................................................... 24
3.5. Fluctuations of the particle charge .................................................................................. 25
4. Electrostatic potential around a particle .................................................................................... 26
4.1. Isotropic plasmas ................................................................................................ 26
4.2. Anisotropic plasmas .............................................................................................. 28
5. Interaction between particles in complex plasmas ........................................................................... 30
5.1. Isotropic plasmas ................................................................................................ 30
5.2. Anisotropic plasmas .............................................................................................. 31
5.3. Experiments .................................................................................................... 31
6. Momentum exchange in complex plasmas ................................................................................. 32
6.1. Momentum transfer cross section ................................................................................... 33
6.2. Momentum exchange rates ........................................................................................ 36
6.2.1. Grain–electron collisions .................................................................................... 37
6.2.2. Grain–ion collisions ........................................................................................ 37
6.2.3. Grain–grain collisions ...................................................................................... 38
6.3. Momentum exchange diagram ..................................................................................... 38
7. Forces on particles in complex plasmas .................................................................................... 40
7.1. Ion drag force ................................................................................................... 41
7.1.1. Binary collision approach ................................................................................... 41
7.1.2. Kinetic approach........................................................................................... 42
7.1.3. Complementarity of the two approaches ....................................................................... 45
7.2. Other forces ..................................................................................................... 45
8. Dynamics of single particles and particle ensembles ......................................................................... 47
8.1. Single particle dynamics .......................................................................................... 47
8.2. Role of charge fluctuations in the particle dynamics.................................................................... 47
8.3. Dynamics of ensembles with spatially varying charges ................................................................. 49
8.4. Complex plasmas as non-Hamiltonian systems........................................................................ 49
9. Waves and instabilities in complex plasmas ................................................................................ 50
9.1. Wave excitation technique ......................................................................................... 50
9.2. Waves in ideal (gaseous) complex plasmas ........................................................................... 51
9.2.1. Major wave modes ......................................................................................... 52
9.2.2. Damping and instabilities of the DIA and DA mode ............................................................. 55
9.3. Waves in strongly coupled (liquid) complex plasmas ................................................................... 58
9.3.1. Longitudinal waves ........................................................................................ 58
9.3.2. Transverse waves .......................................................................................... 60
9.4. Waves in plasma crystals .......................................................................................... 60
9.4.1. One-dimensional string ..................................................................................... 60
9.4.2. Two-dimensional triangle lattice .............................................................................. 61
9.4.3. Three-dimensional plasma crystals............................................................................ 63
9.4.4. Instabilities in plasma crystals ............................................................................... 65
9.5. Nonlinear waves ................................................................................................. 66
9.5.1. Ion solitons and shocks ..................................................................................... 66
9.5.2. Dust solitons and shocks .................................................................................... 66
9.5.3. Mach cones ............................................................................................... 68
10. Phase transitions in strongly coupled complex plasmas ....................................................................... 69
10.1. Strong coupling of dust species..................................................................................... 69
10.2. Phase diagram of Debye–Hückel (Yukawa) systems ................................................................... 71
10.3. Experimental investigation of phase transitions in complex plasmas ...................................................... 72
10.3.1. Melting .................................................................................................. 73
10.3.2. Crystallization............................................................................................. 74
11. Fluid behaviour of complex plasmas ...................................................................................... 76
11.1. Transport properties .............................................................................................. 76
11.2. Hydrodynamic instabilities ........................................................................................ 77
12. Onset of cooperative phenomena in complex plasmas ........................................................................ 79