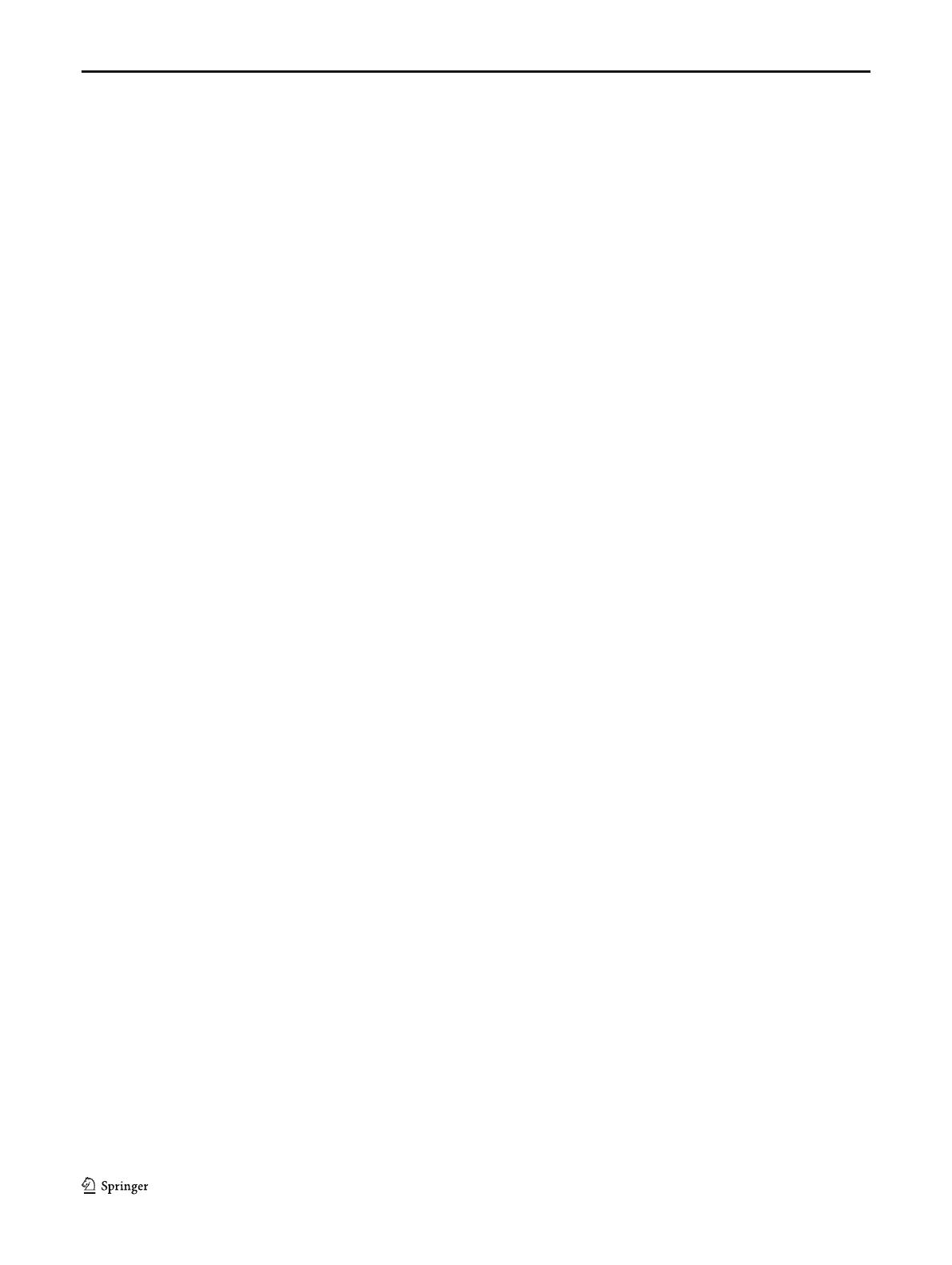
pollution (Lourenço et al. 2011; Iwegbue 2015; Kim et al.
2016; Boughattas et al. 2018; Mkhinini et al. 2020). Mine sites
are generally located adjacent to farmlands and rural areas.
Consequently, these are exposed to pollution by heavy metals,
through air and soil deposits, and additionally, through direct
exposure of the villagers to these pollutants via different path-
ways (Qing et al. 2015; Giri and Kumar 2017; Rezvan et al.
2019).
In this regard, the Pb-Zn mining site of Jebel Ressas and its
surrounding agricultural area, located in northeastern Tunisia
have been abandoned, and huge quantities of mining waste
have been left in the open. The site is considered a source of
heavy metal contamination of farmland surrounding the
mines, especially since no specific management was recorded
up to now to minimize heavy metals contamination (Ghorbel
et al. 2014; Boughattas et al. 2017). Consequently, a health
risk may exist for the habitants due to their exposure to heavy
metal contamination via ingestion, inhalation, and dermal
contact (Ghorbel et al. 2014).
Conversely, poultry raised in the Jebel Ressas mine area
could uptake heavy metals from different origins, mainly
through food. Metal residues may be accumulated on their
tissues and eggs (Chowdhury et al. 2011; Abdulkhaliq et al.
2012). Therefore, trace heavy metals can be transferred to
humans through food consumption (De Vries et al. 2007;
Rodrigues et al. 2012). Béjaoui et al. (2016) affirmed the
health risk for Jebal Ressas inhabitants being exposed to Pb
and Cd contamination through spontaneous plants (Malva
sylvestris) consumption and soil direct dust inhalation.
Considering this, food chain models are crucial tools to
evaluate soil contamination risks. Thus, it is essential to
promote models to describe the pathways related to soil
pollution, dietary transfer of hazardous to animals, and hu-
man exposure from dietary intake of animal products and
plants. The transfer of chemicals in food chains is in gen-
eral detailed by bioaccumulation factors (BAFs) and
bioconcentration factors (BCFs). These key factors are
based on the hypothesis of the existence of linear relation-
ships between heavy metal loads in plants (Helaoui et al.
2020), earthworms (Boughattas et al. 2016), birds (Indrajit
et al. 2018), mammals (Syr-Song et al. 2013;Jebalietal.
2014; Banni et al. 2017), and the amounts existing in soil.
However, the use of constant factors, BCF and BAF, is
controversial since some authors retain it not suitable to
describe the transfer of contaminants from soil to plants
in a particular context (Römkens et al. 2009a,2009b,
Vries et al., 2007). Therefore, more efficient empirical
models have been suggested to depict the soil-plant-
animal transfer. To realistically consider differences in the
bioavailability of trace elements in corresponding to several
soil types, important soil properties (pH, organic matter:
OM, clay) have been considered in such models (Krauss
et al. 2002; Efroymson et al. 2001; Römkens et al. 2009b).
Despite the available literature about the effects of hazard-
ous on ecosystem components, a gap of knowledge still exists
on pollutants transfer modeling from the ecosystem to
humans. This should be underlined in a heavily contaminated
context such as old mining sites.
The objective of the present work is the evaluation of hu-
man risks after exposure to Pb, Cd, Cu, and Zn from the direct
ingestion of Gallus gallus domesticus, which is the most avail-
able and low coast protein source considering the socio-
economic state of the citizens of the Jebel Ressas region.
This evaluation was performed using a predictive modeling
approach considering the supply chain from soil to the final
consumer.
Materials and methods
Study area and sample collection
Jebel Ressas (JR) mine site was considered in this investiga-
tion (Figure S1). JR is located south of the Tunisian capital
“Tunis City”(30 km) and is under a semi-arid climate. The
site includes fields impacted by old mining activities (Pb-Zn
extraction zone).
For the current research, 5 sites were considered:
–Four sites (S1, S2, S3, and S4) with a decreasing
polymetallic contamination gradient in the surrounding
area of the old mine.
–Site 5: Selected as a reference site and located in Chott-
Mariem, in the center of Tunisia (110 km away from the
mining site). We considered this site because it is used for
organic farming with no source of heavy metals pollution
(Figure S1).
Soil samples were collected from the top 0–30 cm of soil in
the different sites (10 samples from each site) after removing
litter and surface vegetation. Earthworms and plants were also
sampled from each site (n=10 from each site).
A total of 100 chickens (20 from each site) were raised for 6
months (March and July 2017). Soil macro-flora including
earthworms and spontaneous vegetation were their main food
diet. Before their sacrifice, chickens were weighed, and then
they were dissected. All the experimental protocols were car-
ried out in accordance with the principles and recommenda-
tions of Directives 86/609/EEC regulating the welfare of lab-
oratory animals (Louhimies 2002; Elkribi-Boukhris et al.
2020). The liver, kidney, lung, and muscle were carefully
removed. Additionally, the blood from the scarified chicken
was sampled. All the samples were then conserved at −80°C
for further analysis. Fresh eggs were collected (20 eggs from
each site) from the five farms that were used for chicken sam-
pling. The eggs were collected and transferred to the
Environ Sci Pollut Res
Content courtesy of Springer Nature, terms of use apply. Rights reserved.