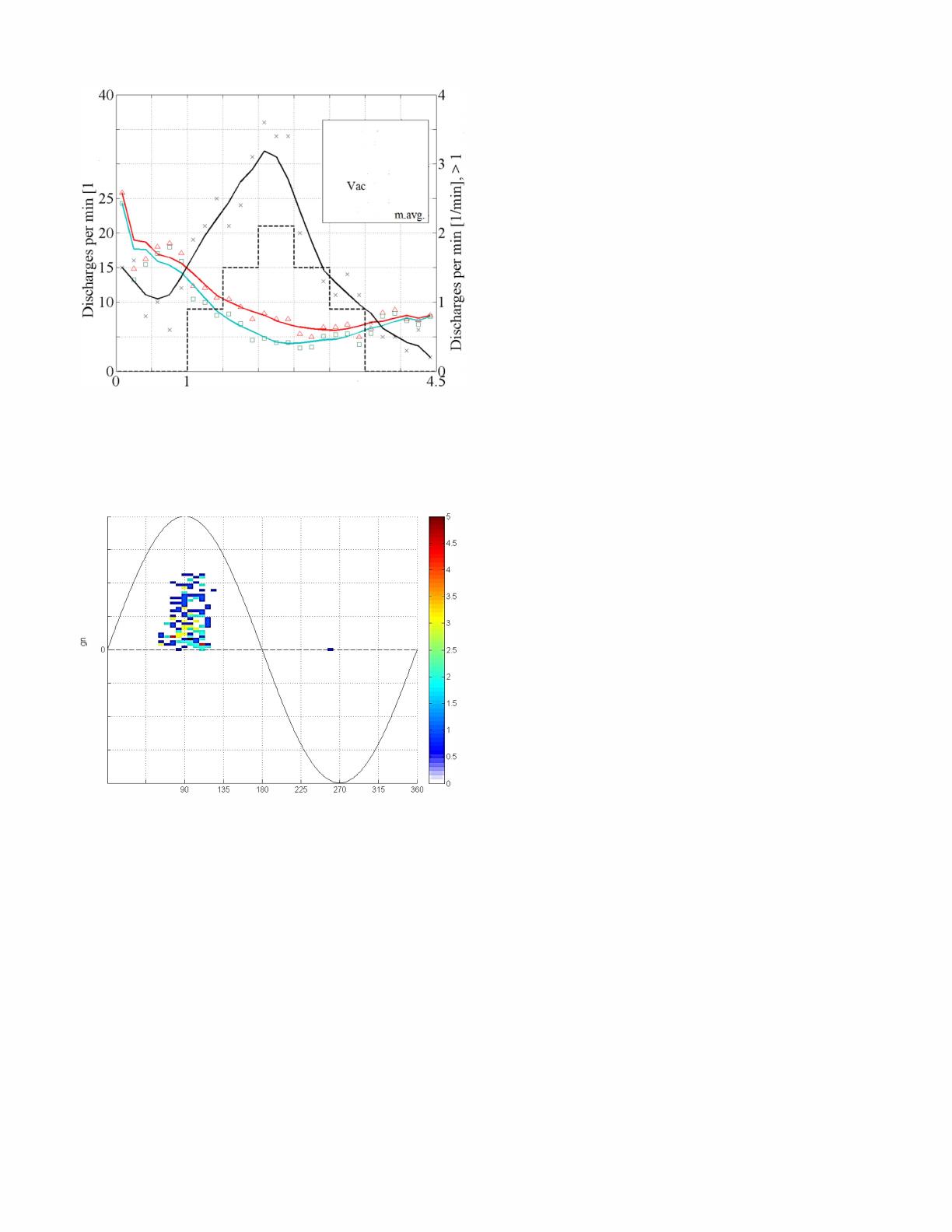
๎
๎ท
๎ฉ๎ช๎ซ๎ฌ๎ญ๎ฎ๎ฏ๎ฐ๎ฑ
๎๎
๎๎๎
๎ค๎ฆ๎๎ท๎
๎ฅ๎ฆ
๎๎
๎๎๎๎น ๎๎๎๎น ๎๎ ๎๎๎๎
๎๎๎ง๎๎๎พ๎๎๎น
๎๎ ๎จ๎๎ฒ๎ฐ๎
๎๎๎ผ๎๎ณ๎ฑ๎๎๎๎ฉ๎ฎ๎ฎ๎ฟ๎ช๎ผ
๎๎ผ ๎
๎ผ๎๎๎ธ๎๎ฒ๎ฒ๎
๎๎๎ผ๎๎๎ธ๎๎ณ๎ณ๎๎๎๎ฌ๎ฎ๎ฎ๎๎ซ๎ผ
๎๎๎๎๎จ๎ผ
๎ฅ
๎ญ๎ถ๎ผ๎น๎ฝ๎๎ฑ๎
๎ฐ๎ผ ๎๎ผ๎๎๎ธ๎ณ๎ณ๎
๎๎๎ผ ๎พ๎๎ต๎๎ฐ๎ฏ๎๎๎จ๎๎๎๎๎๎
๎๎ ๎๎๎๎น ๎๎น
๎๎
๎ถ
๎๎ผ
๎๎ผ
๎๎ ๎ด๎
๎บ๎ ๎๎๎ท๎๎๎ก๎๎๎ง๎๎ฉ๎
๎๎๎ง๎๎๎น๎ง๎๎ฅ๎๎ซ๎๎ซ๎๎ฃ๎๎น๎ง๎๎ซ๎๎น๎๎ฃ๎ง๎น๎๎๎ฉ๎
๎๎๎ง๎๎๎ฉ๎น๎๎ฃ๎ง๎น๎๎๎๎
๎น๎๎๎ฃ๎ฐ๎๎น
๎๎๎๎น๎๎๎๎ฃ๎ฑ๎น๎๎๎๎น๎ฅ๎๎๎น๎๎๎น๎๎น๎น๎ง๎ฅ๎น๎๎๎๎น๎๎๎๎
๎น๎๎๎๎น๎๎๎๎น๎๎๎๎น๎๎๎ฌ๎บ๎๎๎๎ซ๎น
๎๎๎ท๎ฅ๎๎น ๎ถ๎๎๎ฅ๎๎๎๎๎น๎๎๎ซ๎๎ง๎ฐ๎๎๎น๎๎ฉ๎น๎๎๎น๎๎๎๎๎น๎ท๎๎๎น๎ด๎๎๎ณ๎๎ฉ๎น๎ฃ๎๎น๎ซ๎๎๎น๎๎๎๎น๎๎๎ฐ๎๎น
๎ซ๎๎๎น๎ง๎๎ฅ๎๎ซ๎๎ซ๎๎ฃ๎๎น๎ง๎๎ซ๎๎น๎๎ฃ๎ง๎น๎๎๎ฉ๎
๎๎๎ง๎๎๎ฉ๎น๎๎๎ฃ๎ฐ๎๎น๎๎๎๎น๎ฅ๎๎๎น
๎๎ณ๎ณ๎ผ
๎ฝ๎ณ๎ณ๎ผ
๎บ๎ณ๎ณ๎ผ
๎ค๎ผ
๎พ๎ผ ๎ท๎ณ๎ณ๎ผ
๎๎
๎๎
๎ง๎น๎ผ
๎๎
๎๎
๎๎
๎ข๎
๎๎๎๎ท๎ด๎ณ๎ผ
๎๎ผ
๎๎ป๎ณ๎ณ๎ผ
๎๎ฝ๎ณ๎ณ๎ผ
๎๎๎ณ๎ณ๎ด๎ผ ๎ฟ๎๎ผ ๎๎๎ฏ๎ฅ๎บ๎ผ๎๎๎ฅ๎๎ฆ๎๎๎๎ผ๎ซ๎๎บ๎๎ฌ๎ผ
๎บ๎ ๎๎๎ท๎๎๎ฃ๎๎๎ง๎๎ฉ๎
๎๎๎ง๎๎๎น๎๎๎๎ฉ๎๎ซ๎ด๎น๎ฅ๎๎ฃ๎ซ๎น๎ฐ๎๎ง๎ฉ๎ฎ๎ฉ๎น๎ฅ๎๎๎ฉ๎๎น๎๎๎๎๎๎น๎ฃ๎๎น๎ซ๎๎๎น๎ข๎ฅ๎น
๎ฐ๎ฃ๎๎ซ๎๎๎๎๎น๎ฐ๎๎๎ฉ๎ฎ๎ง๎๎๎น๎๎ซ๎น๎๎๎น๎๎น๎น๎ง๎ฅ๎น๎
๎๎๎๎๎น๎๎๎ฌ๎บ๎๎ข๎ฅ๎
๎น๎
๎ฃ๎๎ฃ๎ง๎น๎๎๎ง๎น
๎ฉ๎๎ฃ๎ฑ๎ฉ๎น๎๎ฎ๎๎๎๎ง๎น๎ฃ๎๎น ๎๎๎ฉ๎
๎๎๎ง๎๎๎ฉ๎น๎ฃ๎
๎
๎ฎ๎ง๎ง๎๎๎๎น๎๎ฎ๎ง๎๎๎๎น๎๎๎น๎๎๎๎ฎ๎ซ๎๎ฉ๎น๎ฃ๎๎น
๎๎ฅ๎ฅ๎๎๎๎๎น๎ฐ๎ฃ๎๎ซ๎๎๎๎๎น
๎๎๎ ๎๎๎ ๎๎ข๎ ๎ ๎๎๎๎
๎ท๎๎๎น ๎ง๎ฅ๎น ๎๎๎๎
๎๎๎๎ง๎๎น ๎๎๎๎ฉ๎ฎ๎ง๎๎๎๎๎ซ๎ฉ๎น ๎๎๎น ๎ช๎๎๎ฎ๎ง๎๎น ๎๎น ๎ฉ๎๎ฃ๎ฑ๎น ๎๎น ๎๎๎๎๎น
๎ง๎๎ฅ๎๎ซ๎๎ซ๎๎ฃ๎๎น๎ง๎๎ซ๎๎น๎๎ฎ๎ฉ๎ซ๎น๎๎๎๎ง๎น๎ซ๎๎๎น๎ง๎ฅ๎น๎ฐ๎ฃ๎๎ซ๎๎๎๎น๎๎ฉ๎น๎ซ๎ฎ๎ง๎๎๎๎น๎ฃ๎๎
๎น๎๎๎๎น๎๎น๎ง๎๎ฅ๎๎๎น
๎๎๎
๎ง๎๎๎ฉ๎๎น ๎๎๎๎ฃ๎ง๎๎น ๎๎น ๎ฉ๎ซ๎๎๎๎๎น ๎ฅ๎๎๎ซ๎๎๎ฎ๎น ๎๎ฉ๎น ๎ง๎๎๎
๎๎๎๎๎น ๎ท๎๎๎น ๎๎๎ฉ๎
๎๎๎ง๎๎๎ฉ๎น
๎๎๎๎ฃ๎ฑ๎น ๎๎๎๎น๎ฅ๎ฅ๎น ๎ฉ๎ซ๎๎๎๎๎๎ถ๎๎น ๎๎๎ฉ๎ซ๎๎ง๎น ๎๎๎๎น ๎ฃ๎
๎
๎ฎ๎ง๎น ๎๎๎ฃ๎ฎ๎ซ๎น ๎๎๎น ๎ซ๎๎๎๎ฉ๎น ๎๎ฃ๎ง๎๎น
๎ฃ๎๎๎๎น๎ซ๎๎๎๎น๎ซ๎๎๎น๎๎๎ฉ๎
๎๎๎ง๎๎๎ฉ๎น๎๎๎ฃ๎ฐ๎๎น๎๎๎๎น๎ฅ๎ฅ๎๎น ๎ข๎ซ๎น๎
๎ฃ๎๎๎๎๎๎๎น ๎ฐ๎ฃ๎๎ซ๎๎๎๎
๎น
๎ฉ๎๎ฃ๎ฑ๎๎น๎๎๎น๎ช๎๎๎ฎ๎ง๎๎น๎๎
๎น๎ซ๎๎๎น๎๎๎ฉ๎
๎๎๎ง๎๎๎ฉ๎น๎ฎ๎๎๎๎ง๎น๎๎๎๎น๎ฅ๎ฅ๎น๎๎๎ฐ๎๎น๎๎น๎ฉ๎๎๎๎๎ซ๎๎ด๎น
๎๎ฃ๎ฑ๎๎ง๎น๎ง๎๎ฅ๎๎ซ๎๎ซ๎๎ฃ๎๎น๎ง๎๎ซ๎๎น๎ซ๎๎๎๎น๎ฎ๎๎๎๎ง๎น๎ฅ๎ฎ๎ง๎๎น๎ง๎ฅ๎
๎น๎๎ฎ๎ซ๎น๎ซ๎๎๎น๎๎๎ฉ๎
๎๎๎ง๎๎๎ฉ๎น๎ฃ๎ฐ๎๎ง๎น
๎๎๎๎น๎ฅ๎ฅ๎น๎๎๎
๎ง๎๎๎ฉ๎๎น๎๎๎๎๎๎ง๎๎ด๎น๎ฑ๎๎ซ๎๎น๎ซ๎๎๎น๎๎ฅ๎ฅ๎๎๎๎๎น๎ข๎ฅ๎น๎ฐ๎ฃ๎๎ซ๎๎๎๎๎น ๎ท๎๎๎น๎ข๎ฅ๎น
๎ฅ๎๎ง๎ซ๎๎๎๎น ๎๎๎ฉ๎
๎๎๎ง๎๎๎น ๎๎๎
๎๎ฅ๎ซ๎๎ฃ๎๎น ๎ฐ๎ฃ๎๎ซ๎๎๎๎น ๎ฑ๎๎ฉ๎น ๎๎๎๎ฉ๎ฎ๎ง๎๎๎น ๎ซ๎ฃ๎น ๎๎๎ฃ๎ฎ๎ซ๎น
๎๎น๎ค๎๎ต๎ฉ๎บ๎๎๎ซ๎๎ฎ๎ฉ๎น๎ซ๎๎๎น๎๎ฅ๎ฅ๎๎๎๎๎น๎ฐ๎ฃ๎๎ซ๎๎๎๎น๎๎ฉ๎น๎๎๎น๎๎น๎ซ๎ฃ๎น๎๎๎น๎๎น๎ฃ๎๎น๎ซ๎๎๎ฉ๎น๎ฐ๎ฃ๎๎ซ๎๎๎๎๎น
๎ข๎
๎
๎ฃ๎ง๎๎๎๎๎น๎ซ๎ฃ๎น๎๎๎๎๎
๎น๎ซ๎๎๎น๎๎๎
๎ง๎๎๎ฉ๎๎น๎๎๎น๎ง๎๎ฅ๎๎ซ๎๎ซ๎๎ฃ๎๎น๎ง๎๎ซ๎๎น๎ฉ๎๎ฃ๎ฎ๎๎๎น๎๎๎น๎๎๎๎๎๎ง๎น
๎๎๎น๎ซ๎๎๎ฉ๎น๎๎ง๎๎๎น๎๎ฉ๎๎๎น๎ช๎๎๎ฎ๎ง๎๎น๎๎๎๎น๎ท๎๎๎น๎๎๎๎ฉ๎ฎ๎ง๎๎๎๎๎ซ๎ฉ๎น๎๎๎ฉ๎ฃ๎น๎ฉ๎๎ฃ๎ฑ๎น๎ซ๎๎๎ซ๎น๎ซ๎๎๎น
๎๎๎ฉ๎
๎๎๎ง๎๎๎น๎๎ง๎๎น๎ฅ๎ฃ๎ฉ๎๎ซ๎๎ฐ๎๎น๎๎๎๎น๎ฃ๎
๎
๎ฎ๎ง๎น๎๎ซ๎น๎ซ๎๎๎น๎ฅ๎ฃ๎ฉ๎๎ซ๎๎ฐ๎๎น๎ฅ๎๎๎๎น๎ฃ๎๎น๎ซ๎๎๎น๎ข๎ฅ๎น
๎ฐ๎ฃ๎๎ซ๎๎๎๎
๎น ๎ฑ๎๎ซ๎๎๎๎น ๎ธ๎๎น ๎๎ฉ๎
๎น ๎ช๎๎๎ฎ๎ง๎๎น ๎๎
๎น ๎๎ฎ๎ซ๎น ๎ฑ๎๎ซ๎๎น ๎๎น ๎๎ฎ๎
๎๎น ๎๎ฃ๎ฑ๎๎ง๎น
๎ง๎๎ฅ๎๎ซ๎๎ซ๎๎ฃ๎๎น๎ง๎๎ซ๎๎น๎ซ๎๎๎๎น๎ซ๎๎๎น๎๎๎ฆ๎ฎ๎๎๎
๎ด๎น๎ฃ๎๎น๎ซ๎๎๎น๎ข๎ฅ๎น๎ฐ๎ฃ๎๎ซ๎๎๎๎๎น
๎ท๎๎๎น๎
๎๎ฐ๎๎ซ๎ด๎น ๎๎๎ด๎น๎ฃ๎๎๎ด๎น๎๎๎น๎ฅ๎๎ง๎ซ๎๎ด๎น ๎๎๎ฉ๎
๎๎๎ง๎๎๎๎น ๎ฟ๎๎๎๎น ๎ท๎๎๎น๎ฅ๎ง๎๎๎๎
๎ซ๎๎๎น
๎๎๎ณ๎๎๎ฎ๎๎น๎๎๎ฉ๎
๎๎๎ง๎๎๎น๎๎๎๎๎๎ซ๎ฎ๎๎๎น๎๎ฉ๎น๎๎๎๎น๎ฅ๎ฅ๎
๎น๎๎ฎ๎ซ๎น๎ซ๎๎๎น๎๎ฃ๎ฉ๎ซ๎น๎๎๎ฆ๎ฎ๎๎๎ซ๎น
๎๎๎ฉ๎
๎๎๎ง๎๎๎ฉ๎น๎๎ง๎๎น๎ฑ๎๎๎๎น๎๎๎๎ฃ๎ฑ๎น๎ซ๎๎๎ฉ๎น๎๎๎๎๎ซ๎๎น๎ท๎๎๎ฉ๎น๎๎๎๎๎ซ๎น๎๎๎น๎๎ณ๎ฅ๎๎๎๎๎๎๎น๎๎ด๎น
๎ฉ๎๎๎๎๎น ๎๎๎ฉ๎
๎๎๎ง๎๎๎ฉ๎น ๎ฃ๎
๎
๎ฎ๎ง๎ง๎๎๎๎น ๎๎ง๎ฃ๎ฎ๎๎๎น ๎ซ๎๎๎น ๎๎๎๎๎ฉ๎น ๎ฃ๎๎น ๎ซ๎๎๎น ๎
๎๎ฐ๎๎ซ๎ด๎น ๎ฃ๎ง๎น
๎ฅ๎๎ง๎ซ๎๎๎๎น๎๎๎ฉ๎
๎๎๎จ๎๎๎น๎ฃ๎๎น๎ซ๎๎๎น๎
๎๎ฐ๎๎ซ๎ด๎น๎ฉ๎ฎ๎ง๎๎๎
๎๎น๎๎ง๎๎๎๎น ๎ญ๎ซ๎น๎๎๎ด๎น๎๎๎ฉ๎ฃ๎น๎๎๎น๎ซ๎๎๎ซ๎น
๎
๎๎๎ง๎๎๎ฉ๎น๎๎ฉ๎น๎๎๎ฅ๎ฃ๎ฉ๎๎ซ๎๎๎น๎๎ซ๎น๎ซ๎๎๎น๎ฃ๎ฅ๎ฅ๎ฃ๎ฉ๎๎ซ๎๎น๎
๎๎ฐ๎๎ซ๎ต๎น๎ฉ๎ฎ๎ง๎๎๎
๎๎
๎น๎ฉ๎๎๎๎๎๎
๎๎๎ซ๎๎ด๎น
๎๎๎
๎ง๎๎๎ฉ๎๎๎๎น ๎ซ๎๎๎น ๎ง๎๎ฉ๎๎๎ฎ๎๎๎น ๎ฐ๎ฃ๎๎ซ๎๎๎๎
๎น ๎ฑ๎๎๎
๎๎น ๎๎๎๎๎๎น ๎ฑ๎๎๎๎น ๎๎๎
๎ง๎๎๎ฉ๎๎น ๎ซ๎๎๎น
๎๎๎๎๎๎ซ๎ฎ๎๎๎น๎ฃ๎๎น ๎ซ๎๎๎น๎ฉ๎ฎ๎๎ฉ๎๎ฆ๎ฎ๎๎๎ซ๎น๎๎๎ฉ๎
๎๎๎ง๎๎๎ฉ๎๎น ๎ท๎๎๎น๎ฑ๎๎๎ซ๎๎๎๎น๎ซ๎๎๎๎น๎๎ฃ๎ง๎น
๎๎ฐ๎๎๎๎๎๎๎๎น ๎๎๎๎
๎ซ๎ง๎ฃ๎๎ฉ๎
๎น ๎๎ฃ๎ฑ๎๎ฐ๎๎ง๎
๎น ๎ฉ๎๎ฃ๎ฎ๎๎๎น ๎๎ฃ๎ซ๎น ๎๎๎ฐ๎๎น ๎๎๎ด๎น ๎๎๎๎๎
๎ซ๎น ๎ฃ๎๎น
๎๎๎ฆ๎ฎ๎๎๎
๎ด๎น ๎๎๎๎น ๎๎๎๎๎๎ซ๎ฎ๎๎๎น ๎ฃ๎๎น ๎ซ๎๎๎น ๎ณ๎ง๎ฉ๎น ๎๎ฃ๎ง๎น ๎๎๎๎ฆ๎ง๎ ๎๎๎ธ๎ป๎๎๎ช๎ด๎๎ ๎ซ๎๎๎น
๎
๎๎๎๎๎๎น๎๎๎น๎ฐ๎ฃ๎๎ซ๎๎๎๎น๎๎ฉ๎น๎๎ฎ๎
๎๎น๎ฉ๎๎ฃ๎ฑ๎๎ง๎น๎ซ๎๎๎๎น๎ซ๎๎๎น๎ฉ๎ซ๎๎ซ๎๎ฉ๎ซ๎๎
๎๎๎น๎ซ๎๎๎๎น๎๎๎๎๎น
๎ซ๎๎๎น๎ซ๎๎๎๎น๎๎๎๎น๎๎ฉ๎น ๎๎๎น ๎ซ๎๎๎น๎ฃ๎ง๎๎๎ง๎น ๎ฃ๎๎น ๎๎๎๎๎๎ฉ๎๎
๎ฃ๎๎๎ฉ๎น ๎ฟ๎๎๎น ๎ฑ๎๎๎๎๎น๎ซ๎๎๎น ๎ซ๎๎๎๎น
๎
๎ฃ๎๎ฉ๎ซ๎๎๎ซ๎น ๎ฃ๎๎น ๎ซ๎๎๎น ๎
๎๎ฐ๎๎ซ๎ต๎น ๎ฐ๎ฃ๎๎ซ๎๎๎๎น ๎ง๎ฅ๎น ๎ฃ๎๎๎ฉ๎๎ซ๎น ๎๎ฉ๎น ๎๎๎น ๎ซ๎๎๎น ๎ฃ๎ง๎๎๎ง๎น ๎ฃ๎๎น
๎ฉ๎๎
๎ฃ๎๎๎ฉ๎๎น
๎น๎ญ๎๎น ๎๎๎๎๎๎ข๎ ๎๎๎๎
๎ท๎๎๎น ๎๎๎๎ฉ๎ฎ๎ง๎๎๎๎๎ซ๎ฉ๎น ๎๎๎๎๎
๎๎ซ๎๎น ๎ซ๎๎๎ซ๎น ๎๎๎ซ๎ง๎ฃ๎๎ฎ๎
๎๎๎๎น ๎๎๎น ๎ข๎ฅ๎น ๎ฐ๎ฃ๎๎ซ๎๎๎๎น
๎ง๎๎ฅ๎ฅ๎๎๎น ๎ฃ๎๎น ๎ซ๎๎๎น ๎ง๎ฅ๎น ๎ฐ๎ฃ๎๎ซ๎๎๎๎น ๎ฑ๎๎๎๎น ๎๎๎
๎ง๎๎๎ฉ๎๎น ๎ซ๎๎๎น ๎ฅ๎๎ง๎ซ๎๎๎๎น ๎๎๎ฉ๎
๎๎๎ง๎๎๎น
๎ง๎๎ฅ๎๎ซ๎๎ซ๎๎ฃ๎๎น๎ง๎๎ซ๎๎
๎น๎๎ฎ๎ซ๎น๎ฃ๎๎๎ด๎น๎๎ฃ๎ง๎น๎ซ๎๎๎น๎๎๎ง๎๎๎ง๎น๎๎๎ฉ๎
๎๎๎ง๎๎๎น๎๎๎๎๎๎ซ๎ฎ๎๎๎ฉ๎๎น๎ท๎๎๎น
๎ง๎๎ฅ๎๎ซ๎๎ซ๎๎ฃ๎๎น ๎ง๎๎ซ๎๎น ๎๎๎๎ฉ๎ฎ๎ง๎๎๎น ๎๎ฉ๎น ๎ฉ๎๎๎๎๎๎
๎๎๎ซ๎๎ด๎น ๎๎ฃ๎ฑ๎๎ง๎น ๎ซ๎๎๎๎น ๎ซ๎๎๎น
๎๎๎ฆ๎ฎ๎๎๎
๎ด๎น๎ฃ๎๎น๎ซ๎๎๎น๎ข๎ฅ๎น๎ฐ๎ฃ๎๎ซ๎๎๎๎๎น๎ท๎๎๎ฉ๎น๎๎ฉ๎น๎๎ฉ๎น๎ฅ๎ง๎๎๎๎
๎ซ๎๎๎น๎ฑ๎๎ซ๎๎น๎ซ๎๎๎น๎ฉ๎๎๎ฅ๎๎๎น
๎ข๎ค๎ฅ๎๎
๎๎ง๎
๎ฎ๎๎ซ๎น๎๎ฃ๎๎๎๎๎น๎ณ๎ง๎๎๎๎๎๎๎๎ง๎ต๎น๎ง๎๎ฉ๎ฎ๎๎ซ๎ฉ๎น๎ฉ๎๎ฃ๎ฑ๎น๎ซ๎๎๎ซ๎น๎๎ฃ๎ง๎น๎๎น๎
๎ฃ๎๎ฉ๎ซ๎๎๎ซ๎น
๎ง๎ฅ๎น๎ฐ๎ฃ๎๎ซ๎๎๎๎
๎น๎๎๎๎น๎๎น๎ฐ๎๎ง๎ด๎๎๎๎น๎ข๎ฅ๎น๎ง๎๎ฅ๎ฅ๎๎๎น๎ฐ๎ฃ๎๎ซ๎๎๎๎น๎๎๎๎ฃ๎ฑ๎น๎๎๎๎น๎ฃ๎๎น๎ซ๎๎๎น
๎ข๎ฅ๎น๎ฅ๎๎ง๎ซ๎๎๎๎น๎๎๎ฉ๎
๎๎๎ง๎๎๎น๎๎๎
๎๎ฅ๎ซ๎๎ฃ๎๎น๎ฐ๎๎๎ฎ๎๎
๎น๎ซ๎๎๎น๎ง๎๎ฅ๎๎ซ๎๎ซ๎๎ฃ๎๎น๎ง๎๎ซ๎๎น๎ฑ๎๎๎๎น๎๎๎น
๎๎๎ฐ๎๎ง๎ฉ๎๎๎ด๎น๎ฅ๎ง๎ฃ๎ฅ๎ฃ๎ง๎ซ๎๎ฃ๎๎๎๎น๎ซ๎ฃ๎น๎ซ๎๎๎น๎ซ๎๎๎๎น๎
๎ฃ๎๎ฉ๎ซ๎๎๎ซ๎น๎ฃ๎๎น ๎ซ๎๎๎น๎๎๎ซ๎๎ง๎๎๎๎น๎๎๎๎น
๎ซ๎๎๎น๎
๎๎ฐ๎๎ซ๎ด๎
๎น๎ข๎๎ผ ๎๎๎๎น๎ฅ๎ง๎ฃ๎ฅ๎ฃ๎ง๎ซ๎๎ฃ๎๎๎๎น๎ซ๎ฃ๎น๎ซ๎๎๎น๎ข๎ฅ๎น๎ฅ๎๎๎๎น๎ฐ๎ฃ๎๎ซ๎๎๎๎๎น
๎๎๎๎๎๎๎๎๎๎ ๎
๎ป๎ฏ๎ผ๎ฃ ๎๎๎๎๎ฅ๎ฆ๎ฐ๎ฅ๎ด๎ฐ๎๎ฅ๎ด๎จ๎๎๎๎๎๎๎๎๎ต๎ด๎ป๎ฅ๎ด๎ฅ๎ธ๎ฐ๎๎๎๎ก๎ฏ๎ฉ๎๎ฉ๎ซ๎ฉ๎ง๎ป๎๎ต๎ช๎๎ฝ๎ต๎ฒ๎ป๎ฅ๎ฎ๎ฉ๎๎จ๎ฐ๎บ๎ป๎ต๎ธ๎ป๎ฐ๎ต๎ด๎๎ต๎ด๎
๎ฅ๎ฎ๎ฉ๎ฐ๎ด๎ฎ๎ ๎ฅ๎ง๎ง๎ฉ๎ฒ๎ฉ๎ธ๎ฅ๎ป๎ฐ๎ต๎ด๎ ๎ต๎ช๎ ๎ฐ๎ด๎บ๎ผ๎ฒ๎ฅ๎ป๎ฐ๎ต๎ด๎ ๎บ๎ฟ๎บ๎ป๎ฉ๎ณ๎บ๎ ๎ผ๎ด๎จ๎ฉ๎ธ๎ ๎ถ๎ฅ๎ธ๎ป๎ฐ๎ฅ๎ฒ๎ ๎จ๎ฐ๎บ๎ง๎ฏ๎ฅ๎ธ๎ฎ๎ฉ๎
๎ฅ๎ง๎ป๎ฐ๎ฝ๎ฐ๎ป๎ฟ๎๎๎๎๎๎๎๎ ๎๎๎๎๎๎ฅ ๎๎๎๎ก๎๎๎ ๎๎๎๎ฅ ๎๎๎๎๎ค๎๎๎๎๎ฅ ๎๎๎๎๎๎ฅ ๎ฝ๎ต๎ฒ๎๎๎๎ค๎๎ฃ๎ถ๎ถ๎๎๎๎๎๎๎๎๎ฃ
๎๎๎๎๎๎ฃ
๎ป๎๎ผ๎ฃ
๎ป๎๎ผ๎ฃ
๎ป๎๎ผ๎ฃ
๎ป๎ก๎ผ๎ฃ
๎ป๎ฃ๎ผ๎ฃ
๎ป๎ค๎ผ๎ฃ
๎ป๎ฆ๎ผ๎ฃ
๎๎๎๎๎๎๎๎ฏ๎ฐ๎ฉ๎ด๎๎ฅ๎ด๎จ๎๎๎๎๎๎๎๎๎๎๎๎ผ๎ง๎ฑ๎ด๎ฅ๎ฒ๎ฒ๎๎๎๎๎ด๎ฅ๎ฒ๎ฟ๎บ๎ฐ๎บ๎๎ต๎ช๎๎ฏ๎ฅ๎ธ๎ณ๎ต๎ด๎ฐ๎ง๎บ๎๎ฐ๎ด๎๎บ๎ผ๎ฆ๎บ๎ฉ๎ฅ๎
๎ถ๎ต๎พ๎ฉ๎ธ๎๎ป๎ธ๎ฅ๎ด๎บ๎ณ๎ฐ๎บ๎บ๎ฐ๎ต๎ด๎๎ง๎ฅ๎ฆ๎ฒ๎ฉ๎บ๎๎ผ๎บ๎ฉ๎จ๎๎ฐ๎ด๎๎ฃ๎ ๎๎๎๎ฃ๎๎๎๎ป๎ธ๎ฅ๎ด๎บ๎ณ๎ฐ๎บ๎บ๎ฐ๎ต๎ด๎๎บ๎ฟ๎บ๎ป๎ฉ๎ณ๎บ๎
๎ต๎ถ๎ฉ๎ธ๎ฅ๎ป๎ฐ๎ด๎ฎ๎ ๎ผ๎ด๎จ๎ฉ๎ธ๎ ๎บ๎ป๎ฉ๎ฅ๎จ๎ฟ๎๎บ๎ป๎ฅ๎ป๎ฉ๎ ๎ง๎ต๎ด๎จ๎ฐ๎ป๎ฐ๎ต๎ด๎บ๎๎๎ ๎๎๎๎๎ฅ ๎๎๎๎๎๎๎๎ ๎๎๎๎๎ฅ ๎๎๎ฅ
๎๎๎ฃ๎๎๎ฅ๎๎๎๎๎ข๎๎๎๎ฅ ๎ฝ๎ต๎ฒ๎๎๎๎๎๎ฃ๎ถ๎ถ๎๎๎๎๎ฆ๎ง๎๎๎๎ง๎ค๎๎ฃ๎๎๎๎ค๎๎ฃ
๎๎๎๎๎ฅ๎น๎ฉ๎บ๎๎ฅ๎ด๎จ๎๎๎๎๎๎ฉ๎จ๎จ๎ฅ๎ธ๎จ๎๎๎๎ฃ๎ต๎ฒ๎ป๎ฅ๎ฎ๎ฉ๎๎ ๎ต๎ผ๎ธ๎ง๎ฉ๎๎๎ต๎ด๎ฝ๎ฉ๎ธ๎ป๎ฉ๎ธ๎๎๎ฃ๎๎๎๎๎ฐ๎ด๎ฑ๎บ๎
๎
๎ก๎ฏ๎ฉ๎๎ ๎ป๎ฅ๎ป๎ฉ๎๎ต๎ช๎๎ป๎ฏ๎ฉ๎๎ญ๎๎ฅ๎ด๎จ๎๎๎บ๎บ๎ผ๎ฉ๎บ๎๎๎ต๎ฐ๎ด๎ฎ๎๎๎ต๎ธ๎พ๎ฅ๎ธ๎จ๎๎๎ ๎๎๎๎๎๎ฅ ๎๎๎๎๎๎๎๎๎๎ฅ
๎ฝ๎ต๎ฒ๎๎๎๎๎๎ฃ๎ถ๎ถ๎๎๎๎๎ฆ๎๎๎๎๎๎๎๎๎๎๎๎๎ฃ
๎๎๎ ๎ฝ๎๎ ๎ฃ๎ฅ๎ด๎ ๎๎ธ๎ผ๎ด๎ป๎๎ ๎๎๎ฏ๎ฟ๎บ๎ฐ๎ง๎บ๎ ๎ฅ๎ด๎จ๎ ๎ง๎ฏ๎ฉ๎ณ๎ฐ๎บ๎ป๎ธ๎ฟ๎ ๎ต๎ช๎ ๎ถ๎ฅ๎ธ๎ป๎ฐ๎ฅ๎ฒ๎ ๎จ๎ฐ๎บ๎ง๎ฏ๎ฅ๎ธ๎ฎ๎ฉ๎ ๎ฅ๎ด๎จ๎
๎ง๎ต๎ธ๎ต๎ด๎ฅ๎๎ ๎๎ฉ๎ง๎ฉ๎ด๎ป๎ ๎ฅ๎จ๎ฝ๎ฅ๎ด๎ง๎ฉ๎บ๎ ๎ฅ๎ด๎จ๎ ๎ญ๎ป๎ผ๎ธ๎ฉ๎ ๎ง๎ฏ๎ฅ๎ฒ๎ฒ๎ฉ๎ด๎ฎ๎ฉ๎บ๎๎๎ ๎๎๎๎๎๎๎ ๎๎๎๎๎ฅ ๎๎๎๎ฅ
๎๎๎๎๎ ๎๎๎๎๎๎ฅ ๎๎๎๎ก๎๎๎ ๎๎๎๎๎ฅ ๎๎๎๎๎ฅ ๎๎๎๎๎๎๎๎ ๎๎๎๎๎ฅ ๎๎๎๎ฅ ๎ฝ๎ต๎ฒ๎๎ ๎๎๎ฃ๎ถ๎ถ๎๎ ๎ค๎ฃ๎๎๎ค๎ฆ๎๎๎ฃ
๎๎ง๎ง๎๎๎ฃ
๎๎๎๎๎จ๎ฏ๎ฐ๎ฑ๎ฅ๎ธ๎ฐ๎๎๎๎๎๎๎๎๎๎ฉ๎ถ๎ฆ๎ผ๎น๎๎๎ฅ๎ด๎จ๎๎๎๎๎๎๎๎ ๎ป๎ฉ๎พ๎ฅ๎ธ๎ป๎๎๎๎๎ต๎ณ๎ถ๎ฅ๎ธ๎ฐ๎บ๎ต๎ด๎๎ต๎ช ๎๎๎
๎ง๎ฏ๎ฅ๎ธ๎ฅ๎ง๎ป๎ฉ๎ธ๎ฐ๎บ๎ป๎ฐ๎ง๎บ๎๎ฅ๎ด๎จ๎ ๎จ๎ฉ๎ฎ๎ธ๎ฅ๎จ๎ฅ๎ป๎ฐ๎ต๎ด๎ ๎ฐ๎ด๎ ๎๎๎ก๎ ๎ฐ๎ด๎บ๎ผ๎ฒ๎ฅ๎ป๎ฐ๎ต๎ด๎๎พ๎ฐ๎ป๎ฏ๎ ๎ฝ๎ฉ๎ด๎ป๎ฉ๎จ๎ ๎ฅ๎ด๎จ๎
๎ผ๎ด๎ฝ๎ฉ๎ด๎ป๎ฉ๎จ๎๎ฝ๎ต๎ฐ๎จ๎บ๎๎๎๎ฐ๎ด๎๎๎๎๎๎ ๎๎๎๎๎๎ฅ๎๎๎๎ก๎๎๎ ๎๎๎๎ฅ๎๎๎๎ฅ๎๎๎๎๎๎๎ ๎๎๎๎ฅ๎๎๎๎๎๎๎๎๎๎ฅ
๎๎๎๎๎๎๎๎๎ฅ๎๎ถ๎ฟ๎ฟ๎๎
๎๎๎ก๎๎๎ฅ๎๎๎๎๎๎ ๎ฅ๎๎๎๎๎๎๎๎๎๎๎ฅ๎๎๎๎ฅ๎๎๎๎ฃ
๎ฏ๎๎ฃ๎ถ๎ถ๎๎๎๎๎๎๎๎๎ค๎๎ฃ
๎๎๎๎ฝ๎๎๎๎๎๎๎๎๎๎ฅ๎ธ๎ป๎ด๎ฐ๎ฑ๎ฅ๎บ๎๎๎๎จ๎๎๎ ๎๎๎๎๎๎๎ฅ ๎๎๎๎๎ก๎๎๎๎๎๎ ๎ฅ ๎๎๎๎ฅ ๎๎๎ ๎๎๎๎๎๎ ๎๎ ๎๎๎๎ฅ
๎๎๎ด๎ฎ๎ฐ๎ด๎ฉ๎ฉ๎ธ๎ฐ๎ด๎ฎ๎๎๎ฐ๎ฉ๎ฒ๎ฉ๎ง๎ป๎ธ๎ฐ๎ง๎บ๎๎๎๎ณ๎ฉ๎ธ๎ฐ๎ง๎ฅ๎ด๎๎บ๎ต๎ง๎ฐ๎ฉ๎ป๎ฟ๎๎ช๎ต๎ธ๎๎ป๎ฉ๎บ๎ป๎ฐ๎ด๎ฎ๎๎ฅ๎ด๎จ๎๎ณ๎ฅ๎ป๎ฉ๎ธ๎ฐ๎ฅ๎ฒ๎บ๎๎
๎๎ง๎ค๎ง๎๎ฃ๎ถ๎๎๎ถ๎ถ๎๎๎๎ฅ๎ฎ๎ฉ๎บ๎๎
๎๎๎ ๎๎ฐ๎ฉ๎ณ๎ฉ๎ฟ๎ฉ๎ธ๎๎ ๎๎๎ ๎ฎ๎ฉ๎ด๎ฉ๎ธ๎ฅ๎ฒ๎ฐ๎๎ฉ๎จ๎ ๎ฅ๎ถ๎ถ๎ธ๎ต๎ฅ๎ง๎ฏ๎ ๎ป๎ต๎ ๎ถ๎ฅ๎ธ๎ป๎ฐ๎ฅ๎ฒ๎ ๎จ๎ฐ๎บ๎ง๎ฏ๎ฅ๎ธ๎ฎ๎ฉ๎
๎ณ๎ต๎จ๎ฉ๎ฒ๎ฐ๎ด๎ฎ๎๎๎๎๎๎๎๎๎๎ ๎๎๎๎๎ฅ๎๎๎๎ฅ๎๎๎๎๎ ๎๎๎๎๎๎ฅ๎๎๎๎ก๎๎๎ ๎๎๎๎๎ฅ ๎๎๎๎๎ฅ ๎๎๎๎๎๎๎๎ ๎๎๎๎๎ฅ
๎๎๎๎ฅ ๎ฝ๎ต๎ฒ๎๎๎๎๎ฃ๎ถ๎ถ๎๎๎ก๎๎๎๎ก๎๎ฆ๎๎ฃ๎๎ง๎ง๎ก๎๎ฃ
๎๎๎๎๎ต๎ธ๎บ๎บ๎ฉ๎ด๎ ๎ฅ๎ด๎จ๎ ๎๎๎ ๎๎จ๎ฐ๎ด๎๎ ๎๎๎ฅ๎ธ๎ป๎ฐ๎ฅ๎ฒ๎๎จ๎ฐ๎บ๎ง๎ฏ๎ฅ๎ธ๎ฎ๎ฉ๎บ๎๎ฐ๎ด๎ ๎ฅ๎ ๎ง๎ฅ๎ฝ๎ฐ๎ป๎ฟ๎ ๎ฅ๎ป๎ ๎ฝ๎ฅ๎ธ๎ฐ๎ฅ๎ฆ๎ฒ๎ฉ๎
๎ฅ๎ถ๎ถ๎ฒ๎ฐ๎ฉ๎จ๎๎ฌ๎ฉ๎ท๎ผ๎ฉ๎ด๎ง๎ฟ๎๎ถ๎ฅ๎ธ๎ป๎๎๎จ๎ฃ๎ณ๎ฉ๎ฅ๎บ๎ผ๎ธ๎ฉ๎ณ๎ฉ๎ด๎ป๎บ๎๎ฅ๎ด๎จ๎ ๎ณ๎ต๎จ๎ฉ๎ฒ๎ฐ๎ด๎ฎ๎๎๎๎๎๎๎๎๎๎ ๎๎๎๎๎ฅ
๎๎๎๎ฅ๎๎๎๎๎ ๎๎๎๎๎๎ฅ๎๎๎๎ก๎๎๎ ๎๎๎๎๎ฅ ๎๎๎๎๎ฅ ๎๎๎๎๎๎๎๎ ๎๎๎๎๎ฅ๎๎๎๎ฅ ๎ฝ๎ต๎ฒ๎๎๎๎ก๎๎ฃ๎ถ๎ถ๎๎๎๎ฃ๎๎๎
๎๎ฃ๎๎ฃ๎๎๎๎๎ฆ๎๎ฃ
View publication statsView publication stats