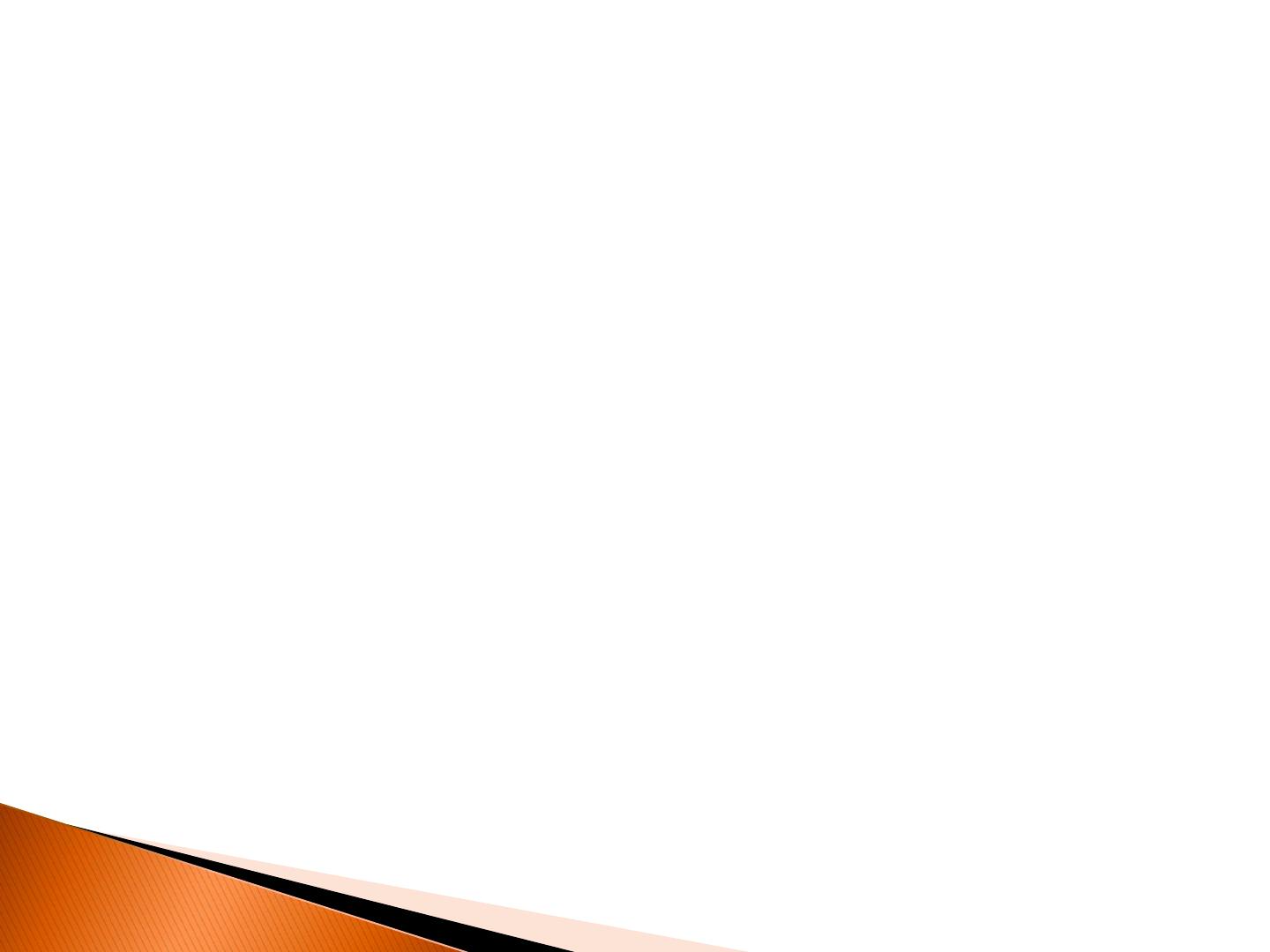
Organization of the practical works
The following practical works (TD) are dealing with basics of industrial
microbiology and fermentation monitoring, which is essentially based
on microbial growth kinetics.
The practical works aim to let you understand the following sections:
1. Microbial nutrition
2. Microbial growth curve and µmax
3. Monitoring microbial growth in culture
At the end of each PW, you will have to prepare a report that will be
given to your teacher (Belaouni Hadj Ahmed), at the end of the
lockdown imposed by the global COVID19 Pandemics.