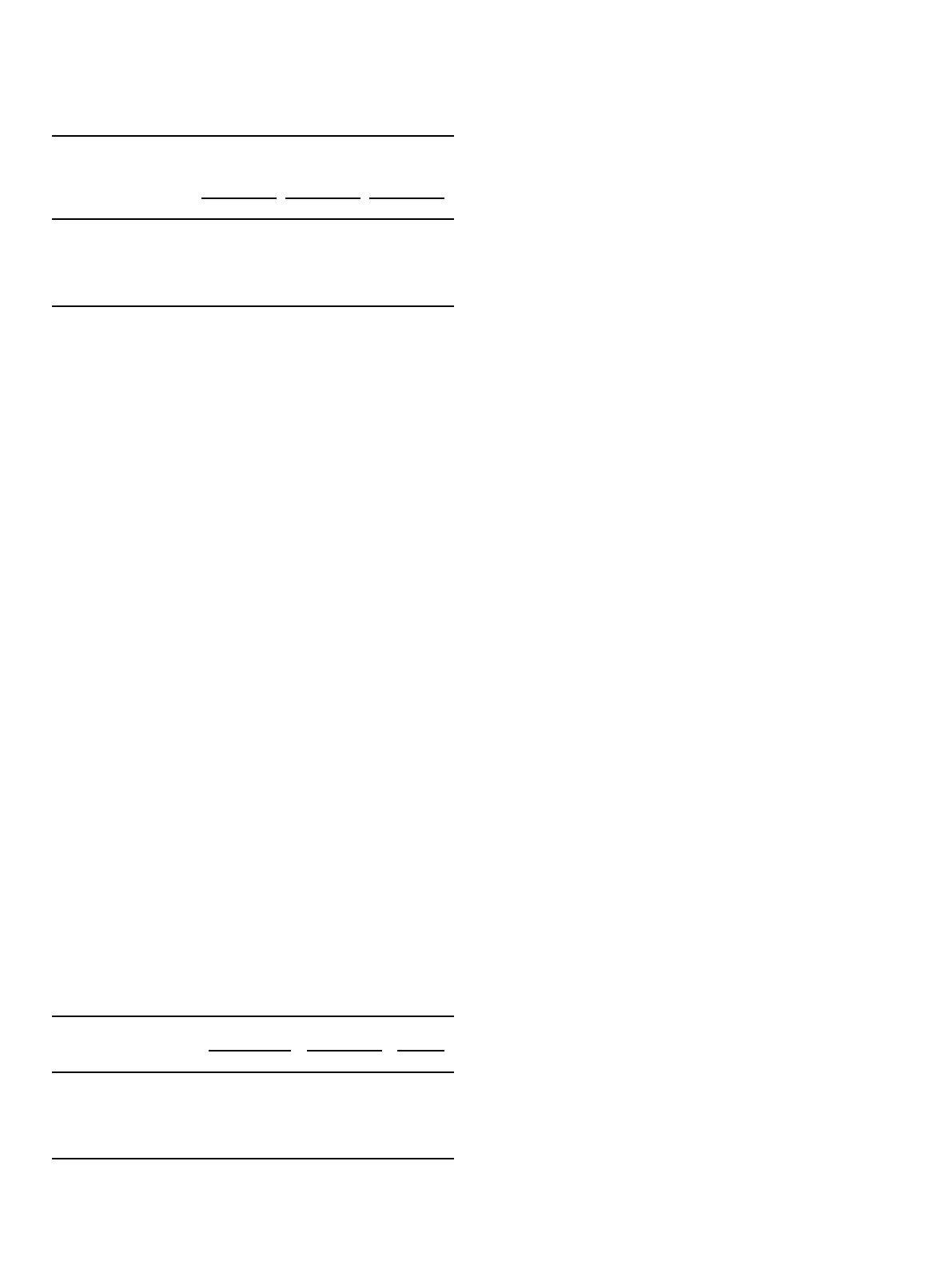
3.4.1. Precocity of the life-cycle
As shown in Table 4, the less advanced species in their larval
stage in May are A. strepens,D.jagoi and C. wattenwilianus, as their
barycenter correspond to an early L2 stage in both stations. In
contrast, A. turrita in its first generation has the more precocious
cycle, as most individuals are L3 in both stations. The two
remaining species are intermediate: the population of A. patruelis is
more advanced in Koléa but the contrary is true for O. harterti.Ifwe
compare the species in both stations, five are in advance (from less
than a week to two weeks) in Koléa. O.harterti is an exception, as
we observe a negative lag of 2e3 weeks in the same station. The
situation in May can be attributed to different components of insect
phenology, such as the speed of larval development and the date of
hatching.
In a first step, we performed a regression analysis on the bary-
centers of dates versus the larval stages, in order to limit the
uncertainty of L1 counting estimation. We also calculated the
elapsed time between the barycenter of L1 and L5 for each station.
As both methods gave comparable results, we show in Table 5 the
times given by this last method, as being easier to interpret. The
fastest larval development is observed in C.wattenwilianus,at
about two months and a half, and the slowest in A. strepens, about
three months. If we compare the populations in both stations, the
insects are divided in two groups: four of which develop faster in
Soumàa than in Koléa, whereas O. harterti and A. turrita take less
time to accomplish their larval life in Koléa. Unfortunately, as we
calculated these elapsed times using a two-year survey, we cannot
assess the inter-annual variability. Moreover, as the barycenter of
a given larval stage is calculated at the level of the population, no
statistics can be given.
In a second step, we calculated the barycenter of L1 for each
station and each species (Table 4). Four species begin their larval
life approximately at the same time, between the end of March to
the beginning of April. It seems that C. wattenwilianus is the latest
species to hatch, since L1 individuals are observed by the second or
third week of April. More interestingly, all the species but O. harterti
hatch about two weeks earlier in Koléa than in Soumàa.
3.4.2. Lag in the larval phenology
We calculated for each year (1991 and 1992) the lag in the larval
development between both stations (Table 5). The Wilcoxon tests
conducted with the data set of the two years taken together show
that the larval cycle is about one to two weeks (A. strepens,first
generation of A. turrita) later in Soumàa than in Koléa. In contrast,
there is an opposite shift in O. harterti phenology, the population of
Soumàa developing 1e
3 weeks in advance, relatively to the Koléa
population. The life cycles of the three remaining studied species
(A. patruelis,C. wattenwylianus and D. jagoi jagoi) show non-signifi-
cant lags.
To summarize, simultaneous onset of the adult stage in two
species can result from opposite strategies: early hatching and slow
larval development or late hatching and fast development.
However, even if the statistical support is weak, four species show
a more or less advanced phenology in Koléa relative to Soumàa.
O. harterti, in its first generation, is a noticeable exception as it
presents an opposite trend.
4. Discussion
4.1. Seasonal structured assemblages
The inventory of the insect species in the two stations revealed
a relatively rich orthopteran fauna as 28 species were recorded at
the station of Koléa, 24 at the station of Soumàa.
Samplings were done in two waste lands similar in their plant
composition and covering (78% of shared plant species). As for
insect inventories, both methods used (CAH and ANOSIM) show
that three seasonal assemblages of Orthoptera can be defined, the
richest, developed in summer-autumn, being differentiated at the
two studied stations.
As to the possible factors driving these differences, several
hypotheses can be brought: (i) vegetation composition or structure
(Agabiti et al., 2006; Dufrêne and Legendre, 1997; Guido and
Gianelle, 2001; and Vassiliki et al., 2003), (ii) historic reasons,
such as a different interval since perturbation occured (fire for
example, see Langlands et al., 2006), (iii) more or less pronounced
grazing pressure by goat and sheep (Louveaux et al., 1996; Feoli
et al., 2002; Fadda et al., 2008), and (iv) climatic parameters. Of
course, the diversity of the studied sites depends on the diversity of
the areas surrounding them, particularly the existence of corridors,
but such information is still lacking. As the history of both studied
sites is, as far as we know, quite similar, we considered the climatic
parameters as the main explanation of differences between sum-
mereautumn assemblages, especially for the less abundant species.
Analysis of climatic data in both stations was revealed as
complex by several considerations. First, the dry season appears in
June in Koléa, but earlier in May in Soumàa. Second, the mean
temperatures are one to two degrees higher in Soumàa than in
Koléa. However, we recorded more extreme temperatures in Sou-
màa, due to the more significant distance between this locality and
the Mediterranean Sea. As a result, the minima temperatures
recorded are lower in Soumàa than in Koléa, particularly in winter.
Taken together, several more or less contradictory predictions
about the studied insects can be advanced in such a situation.
- At the life cycle level, one can expect two contrasting reactions
of the species
- A faster transition between larval stages in the station char-
acterized by higher mean temperatures (Soumàa): a question
of plasticity.
- An earlier emergence of adults in the station where the dry
period is recorded earlier (Koléa): a question of local
adaptation.
Table 4
Precocity of the life cycle.
Species name Mean
barycenter of
larval stages in
May
Elapse time
between L1-L5
(in month)
Mean
barycenter of
L1 (in month)
Koléa Soumàa Koléa Soumàa Koléa Soumàa
Acrotylus patruelis 2.81 2.28 3.09 2.87 3.91 4.13
Aiolopus strepens 2.43 2.11 3.24 2.95 3.93 4.33
Dociostaurus jagoi 2.26 2.14 3.14 2.74 4.00 4.20
Calliptamus wattenwilianus 2.29 2.2 2.52 2.25 4.54 4.75
Ochrilidia harterti G1 2.39 3.04 2.67 3.33 4.14 3.25
Acrida turrita G1 3.38 3.33 2.72 3.38 3.50 4.17
A barycenter of 2.81 in May corresponds to a mean stage closer to L3 than to L2. A
mean barycenter of L1 of 3.91 indicates a month mode closer to April than to March.
Table 5
Lag in the larval phenology in dominant species between Koléa and Soumàa.
Species name Number of
larvae recorded
Lag p-value
Koléa Soumàa 1991 1992 Wilcoxon
Acrotylus patruelis 113 119 0.64 0.25 0.48 NS
Aiolopus strepens 286 189 0.54 0.24 0.016 *
Dociostaurus jagoi 252 183 0.08 0.13 0.19 NS
Calliptamus wattenwilianus 73 39 0.02 0.08 0.40 NS
Ochrilidia harterti G1 192 224 0.72 0.19 0.028 *
Acrida turrita G1 99 42 0.51 0.20 0.067
A0.40 mean lag indicates that the phenology of larva stages is 0.4 month later in
Soumàa than in Koléa.
A. Guendouz-Benrima et al. / Journal of Arid Environments 75 (2011) 416e423420