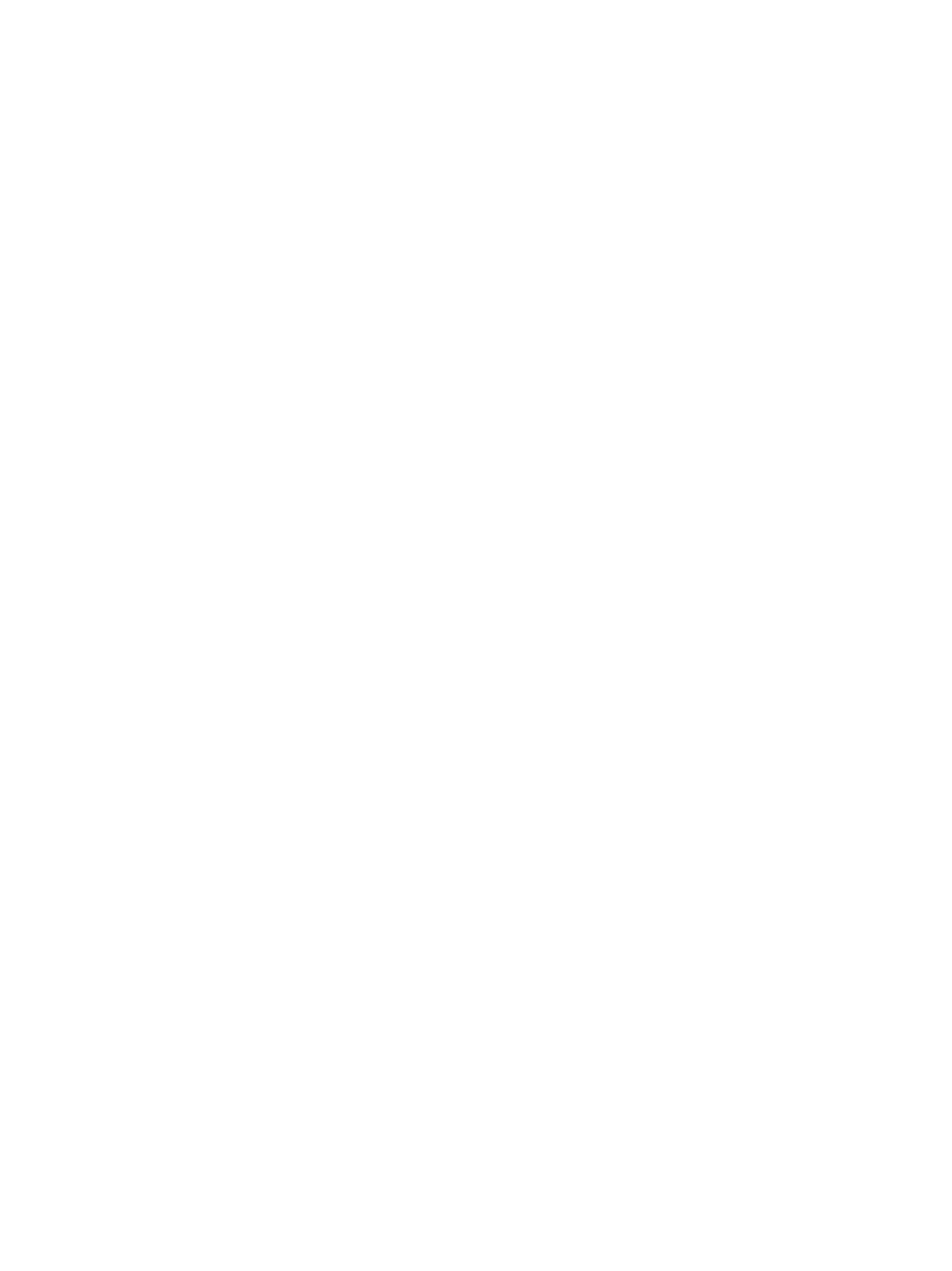
M.-W. Lu et al. / Molecular Immunology 45 (2008) 1146–1152 1147
rhabdovirus (SHRV) which was shown to cause mortalities
exceeding 40% in zebrafish (Phelan et al., 2005).
In view of the differential effect of viral infection, we looked
into the zebrafish interferon response, in which the zebrafish
interferon gene (zIFN) has been recently identified as to having
anti-virus function (Altmann et al., 2003) and may contribute to
both induction and regulation of the innate and adaptive immu-
nity. Downstream interferon activated Mx gene has also been
identified in zebrafish, grouper, salmon, trout, and halibut upon
infection with aquatic viruses (Chen et al., 2006; Kibenge et
al., 2005; Lin et al., 2005; McBeath et al., 2006) suggesting
the importance of the interferon regulatory pathway including
RNA-activated protein kinase (PKR) and the 2-5A proteins dur-
ing viral infection. In our present study, we have observed an
elevated interferon expression in infected adult zebrafish rela-
tive to infected larvae resulting in higher rate of mortality in the
latter. This may indicate that the susceptibility to NNV infection
is dependent on the enhancement of IFN system. However, the
mechanism in which interferon response is activated upon NNV
viral infection needs to be further elucidated.
2. Materials and methods
2.1. Fish
AB (−) inbred strain zebrafish were obtained at 2 months
stage from Institute of Cellular & Organismic Biology,
Academia Sinica, Taiwan. The zebrafish were handled according
to Institutional Animal Care and Use Committee guidelines.
2.2. Cell lines and virus
The SSN-1 derivate cell line E-11 (Iwamoto et al., 2000)
was used to isolate and titrate NNV. Cells were propagated and
maintained at 28 ◦C in L-15 medium (GIBCO) supplemented
with 10% fetal bovine serum (Sigma), 100 I.U./ml penicillin,
and 0.1 mg/ml streptomycin.
The nodavirus used in this study was isolated from mal-
abaricus grouper (Epinephelus malabaricus) juveniles during
an outbreak of VER at a fish farm in southern Taiwan. Sequence
analysis of partial viral genome (data not shown) revealed that
it shares more than 95% homology with other betanodaviruses
isolated from fish in Taiwan (Chi et al., 2003). E-11 cells were
inoculated with a fourth passage virus supernatant and incubated
at 28 ◦C. When a cytopathic effect (CPE) was observed on 3 days
post-infection (p.i.), the culture supernatant was harvested, clar-
ified by centrifugation (3000 ×gfor 5 min) and stored at 4 ◦C
until challenge. The virus titer in the supernatant was determined
by using an infectivity assay and was calculated to be 1 ×108
TCID50 ml−1.
2.3. Fish challenge
Two groups of 60 adult zebrafish, one infected and one mock-
infected, were used in the challenge experiment. The fish were
held in 5 liter tanks at a water temperature of 28 ◦C. Chal-
lenge was performed by intraperitoneal (i.p.) injection of 1 ×105
TCID50 ml−1of NNV in 20 l. The mock-infected group was
injected with PBS. After challenge, the fish were monitored daily
over a 14-day period for signs of disease and mortality. Six fish
in each group were sampled each day and tissue samples from
different organs were isolated for virus detection by RT-PCR and
infectivity assay (TCID50). In a separate experiment, fish were
maintained and challenged similarly, but three fish from each
group were sampled daily for histology and immunohistochem-
istry. Some fish in parallel were also collected for confirmation
of infection by RT-PCR and virus re-isolation.
2.4. Histology and immunohistochemistry (IHC)
The heads of fish used for histology were fixed for at
least 24 h in neutral phosphate-buffered 10% formalin and
embedded in paraffin wax. Five micrometers brain sections
were stained with haematoxylin and eosin (H&E) whereas the
other parallel sections were processed for immunohistochemical
detection of nodavirus protein using anti-NNV antiserum and
a streptavidin-biotin-alkaline-phosphatase complex antibody
detection technique (Chemicon IHC Select Kit). Mock-infected
fish served as negative controls for the experiment.
2.5. RNA isolation and RT-PCR
RNA was extracted using TRIZOL reagent (Life Technolo-
gies) according to the manufacturer’s instructions. The RT-PCR
was performed using a Superscript III one-step RT-PCR sys-
tem (Invitrogen) and each reaction includes 2 l total RNA
(20 ng) and a primer set. The primers were designed to amplify
the variable T4 region of the coat protein gene (Nishizawa
et al., 1994), Mx (sense 5-AGTACCGGGGAAGAGAGCT-
A-3antisense 5-AAGGTGGCATGATTGT CTGT-3), IFN
(sense 5-ATGAGAACTCAAATGTGGAC-3antisense 5-TT-
ACA CTCGAGGATTGAC-3), and -actin (sense 5-ATGGAT-
GAGGAAATCGCTG-3antisense 5-ATGCCAACCATCA-
CTCCCTG-3) gene.
2.6. Microinjection of virus into zebrafish larvae
The NNV titer for microinjection was adjusted to 1 ×103
TCID50 ml−1. Injections were conducted by PLI-100 air-
injection apparatus (Medical System Co.). The amount
(approximately 0.1 l) injected into larvae was estimated by
visualizing the injection volume. After injection, the larvae were
incubated in the Petri-dish at 28 ◦C.
2.7. Immunoblot
Fish samples were collected, homogenized, and solubilized
in disruption buffer containing 50 mM Tris–HCl (pH 7.0), 5%
2-mercaptoethanol, 2% sodium dodecyl sulfate (SDS), and
2.75% sucrose. Samples were then sonicated, boiled, sub-
jected to electrophoresis on denaturing 12% polyacrylamide
gels, transferred to nitrocellulose membranes, blocked with
5% nonfat milk, and reacted with antibodies against phospho-