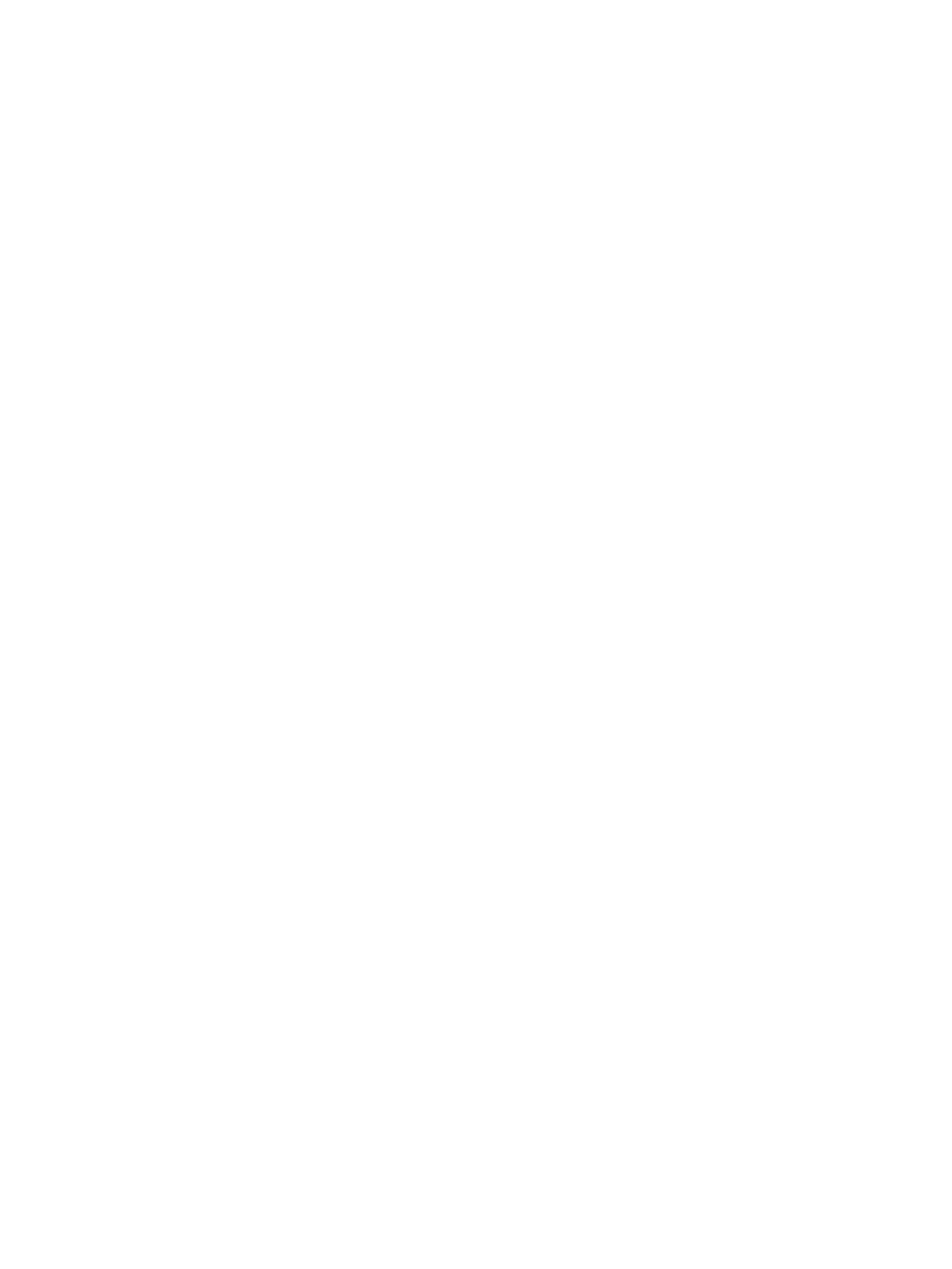
derivatized with o-phthaldialdehyde and separated by isocratic
reversed-phase chromatography on a Symmetry C18 column (150 ×
4.6 mm, 5 μm particle size; Waters Corp., Milford, MA, USA). Potassium
phosphate buffer (50 mM, pH 6.6) containing 12% v/v acetonitrile was
used as the mobile phase at a flow rate of 1.1 ml/min and a column tem-
perature of 35 °C. Fluorescence detection was performed at the excita-
tion and emission wavelengths of 340 and 450 nm, respectively.
The serum activities of ALT and AST and the concentration of protein
in the homogenates were assayed with commercial methods in a certi-
fied laboratory.
Total protein concentrations in supernatants of homogenates were
assayed with commercial methods in a certified laboratory on the Di-
mension RxL-Max apparatus on Flex kit. Briefly, cuprum-cation inter-
acts with a peptide bond in protein in an alkaline solution; the
amount of Cu(II) complex with blue color, proportional to protein con-
centration, is measured using bichromatic technique of final point
assessment.
2.6. Statistical analysis
Data were expressed as the mean values ± SD. Statistical analysis of
the effect of the age and IR on iNOS level, as well as DDAH and amino-
transferases activity, was performed using a two-way analysis of vari-
ance (ANOVA). Statistical analysis of the effect of age and the time of
reperfusion on Arg, ADMA, and SDMA levels and Arg/ADMA (A/A)
ratio was performed using MANOVA with repetition. Specificcompari-
sons were made using contrast analysis. The hypotheses were consid-
ered positively verified if p b0.05.
3. Results
3.1. ADMA, SDMA, Arg, and A/A ratio
The concentration of ADMA measured before IR was comparable in
all groups of rats regardless of age. At 15 min of reperfusion in the IR-
2 group the increase in ADMA concentration was observed and the dif-
ference between that group and the C-2 group was significant (IR-2 vs.
C-2, p b0.05). Otherwise, the concentration of ADMA was significantly
decreased in the ischemic group of the older rats (IR-12 vs. C-12,
pb0.05). Those changes in the ischemic groups were the reason for
the significant difference between young and older ischemic groups
(IR-2 vs. IR-12, p b0.001). At 120 min of reperfusion the concentration
of ADMA in the IR-12 group slightly increased and achieved comparable
level as in non-ischemic groups. At this point in time, the concentration
of ADMA in the IR-2 group was still the highest of all the groups (IR-2 vs
C-2, p b0.05; IR-2 vs. IR-12 and C-12, p b0.005 in both comparisons).
In 240 min of reperfusion, the level of ADMA decreased in all the
groups. However, it was still significantly higher in the IR-2 group
than in group of older ischemic rats (IR-2 vs. IR-12 and C-12, p b0.05
in both comparisons). No significant differences between the C-2 and
the C-12 groups in all time points were observed (C-2 vs. C-12,
p=NS)(Fig. 1A).
Concentrations of SDMA were highest in the IR-2 group at all points
of reperfusion. At the end of reperfusion it was significantly higher in
that group compared to the C-12 group (IR-2 vs. C-12, p b0.05) and
was on the border of significance compared to IR-12 (IR-2 vs. IR-12,
p = 0.05) (Fig. 1B).
When analyzing particular time points of reperfusion it was ob-
served that values of A/A ratio changed in the same way as Arg levels.
The initial values of both parameters were significantly higher in the
young groups than in older ones (C-2 vs. C-12, p b0.005 for Arg, C-2
vs. C-12, p b0.05 for A/A ratio as well IR-2 vs. IR-12, p b0.001 for
both parameters). After the IR period the values of those parameters de-
creased at the first 15 min and were significantly lower from that in the
non-ischemic group (IR-2 vs. C-2, p b0.001 for both parameters). At all
points of time (15, 120 and 240 min) the values of Arg level and A/A
ratio were highest in the C-2 group and the differences between that
group and all the others were significant (C-2 vs. IR-2 and IR-12,
pb0.001 for both parameters at all points of time; C-2 vs. C-12,
pb0.05 for Arg at 15 min and p b0.01 for Arg at 120 and 240 min, C-2
vs. C-12 p b0.05 for A/A ratio at all points of time). Differences between
both groups of older rats were also significant. In the non-ischemic groups
of rats Arg level and A/A ratio were higher than in the ischemic ones at all
points of time (C-12 vs. IR-12, p b0.05 for both parameters at 15 min;
p = 0.05 for Arg at 120 min, p b0.01 for Arg at 240 min, and p = 0.07
for A/A ratio at 120 and 240 min). At the end of reperfusion the values
of Arg level and A/A ratio were still highest in the C-2 group. In the groups
of older rats the values of those parameters were higher in the non-
ischemic group than in the ischemic one (C-12 vs. IR-12, p b0.01, for
Arg, and p = 0.07 for A/A ratio) (Fig. 1C, D).
3.2. Biochemical analyses
3.2.1. iNOS
In the C-2 group, iNOS protein concentration was higher than in the
C-12 group and the difference between those groups was on the border
of significance (C-2 vs. C-12, p = 0.056). The increase in iNOS protein
level was observed in the IR-2 group compared to the C-2 group (IR-2
vs. C-2, p b0.005), which was not revealed in the groups of mature an-
imals (C-12 vs. IR-12, p = NS). Therefore, there was a significant differ-
ence between young and older rats subjected to IR (IR-2 vs. IR-12,
pb0.001) (Fig. 2A).
3.2.2. DDAH activity
Activity of DDAH was on a comparable level in the non-ischemic
groups regardless of age. The decrease in DDAH activity was observed
in the IR-2 compared to the C-2 group (IR-2 vs. C-2, p b0.001), which
was not revealed in the groups of older animals (C-12 vs. IR-12,
p = NS). Therefore, there was a significant difference between young
and older rats subjected to IR (IR-12 vs. IR-2, p b0.001) (Fig. 2B).
3.2.3. Aminotransferases activity
Aminotransferases (ALT, AST) activity was higher in the groups of
older rats (C-12, IR-12) than in the young ones (C-2, IR-2) but the differ-
ences between both non-ischemic groups (C-2 vs. C-12, p = NS) and is-
chemic groups (IR-2 vs. IR-12, p = NS) were not significant. The
activity of ALT was significantly higher in the groups subjected to IR
than in the non-ischemic groups regardless of age (IR-12 vs. C-12,
pb0.01 and IR-2 vs. C-2, p b0.05). Similarly, the activity of AST
was significantly higher in the IR-12 than in the C-12 group (IR-12 vs.
C-12, p b0.05), and was on the border of significance in the groups of
young rats (IR-2 vs. C-2, p = 0.08) (Table 1).
4. Discussion
The demand for liver transplantation is continually growing and ex-
ceeding organ availability. It has become a procedure of choice in the
treatment of chronic and acute liver failure. In the USA, more than
3000 liver transplantations are performed annually (Habior et al.,
2002). On the other hand, in today's aging societies, an increasing num-
ber of potential organ donors are found amongst the elderly, so the use
of elderly donors (over 50 years) has significantly increased (Alkofer
et al., 2006; Foster et al., 2007). For our experiment, male Wistar rats
of two different ages, young (2–4 months) and older (12–14 months),
were chosen, which corresponds approximately to a 10- to 15-year-
old age range and an over 50-years-old in humans, respectively (Feng
et al., 2006). A number of distinct age-related alterations have been
identified in the hepatic response to IR, including amongst others, a re-
duced production of antioxidants and a more evident inflammatory re-
sponse (increased expression of pro-inflammatory genes and cytokines
and decreased mRNA expression of antiinflammatory cytokines), a
lower expression of the cytoprotective protein (HSP70), increased
47M. Trocha et al. / Experimental Gerontology 50 (2014) 45–51