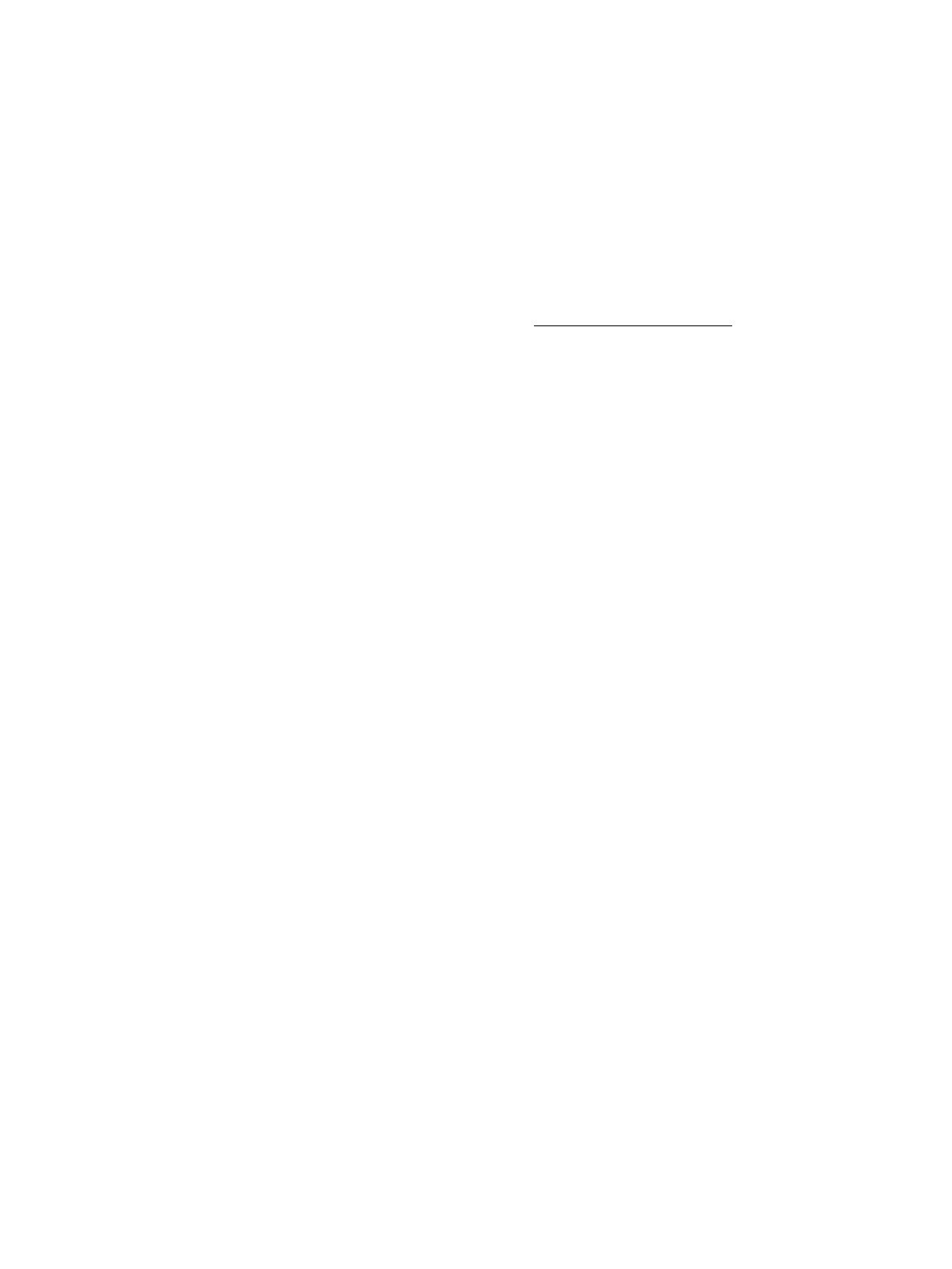
Cissus quadrangularis Linn. belongs to the family Vitaceae and is
indigenous to India, Sri Lanka, Malaysia, Thailand and Africa. The
plant has a great medicinal value and the stem part of C. quadrang-
ularis is traditionally used for the treatment of skin infections, con-
stipation, piles, anemia, asthma, irregular menstruation, burns and
wounds (Kritikar and Basu, 2000). Studies have reported that
methanolic extract from C. quadrangularis (CQEt) have antimicro-
bial, antiulcer and antioxidant properties (Subbu, 1970; Anoop
and Jagdeesan, 2002; Chidambara Murthy et al., 2003). The potent
fracture-healing property of this plant was the first report in 1963
documenting its benefits. Since then, a number of animal studies
have claimed its utility in a wide range of disease conditions like
gastric ulcer, bone fracture and hepatic toxicity (Prasad and Udupa,
1963; Chopra et al., 1975). A recent report shows that CQEt effec-
tively reduces the body weight by inhibiting the oxidation of LDL
cholesterol and by lowering the blood glucose in obese patients
(Oben et al., 2006). It has also been proved that CQEt has therapeu-
tic action against hepatic damage induced by carbon tetrachloride
(CCl
4
)(Jainu and Devi, 2005). However, there is a lack of data on
the protective effects of CQEt on the pathological development of
IR-associated liver disease induced by HFFD.
Metformin, an insulin sensitizer, has become the established
treatment for type 2 diabetes mellitus. Proposed mechanisms for
the antihyperglycaemic effect include enhanced insulin-stimulated
glucose uptake from the blood into the tissues, and decreased glu-
cose production in the liver by suppression of hepatic gluconeo-
genesis (Srividhya and Anuradha, 2002). This study aims to
evaluate the effect of CQEt on HFFD-induced fatty liver and IR
and compare the data with that of metformin. Additionally, a sys-
tematic evaluation of its free-radical scavenging property of CQEt is
carried out in vitro.
2. Materials and methods
2.1. Preparation of plant extract
The fresh stem of C. quadrangularis was purchased from the local market, at
Chidambaram, washed, cut into pieces and sun-dried. The dried stems were finely
powdered. One kilogram of the dried plant material was exhaustively extracted
with 2 L methanol. The extract was filtered and distilled on a water bath. The crude
extract was vacuum dried and powdered (Jainu and Devi, 2005). The yield of the ex-
tract was 4.8 g% .The powder was mixed in the diet for in vivo studies and dissolved
in saline for in vitro studies.
2.2. Animals and treatment protocol
Adult male Wistar albino rats weighing 150–170 g were obtained from the Cen-
tral Animal House, Rajah Muthiah Medical College, Annamalai University. The ani-
mals were housed in large polypropylene cages in a temperature-controlled room
and provided with standardized pelleted feed (Amrut rat and mice feed, Bangalore,
India) and clean drinking water ad libitum. All the experimental procedures were
carried out in accordance with the guidelines of the Institutional Animal Ethics
Committee (IAEC).
After acclimatization a period of one week, the animals were divided into two
groups and fed the control or HFFD. After 15 days, IR was confirmed by measuring
fasting glucose and insulin and by computing fasting insulin sensitivity indices. On
day 16, the rats in each group were divided treated with CQEt, metformin or left un-
treated. Accordingly five groups of rats were maintained for 60 days as follows:
Group I – CON – normal diet-fed rats.
Group II – HFFD-fed rats.
Group III – HFFD + CQEt – HFFD-fed rats treated with CQEt (10 g/100 g diet).
Group IV – HFFD + MET – HFFD-fed rats treated with metformin (50 mg/kg/
day).
Group V – CON + CQEt – normal diet-fed rats treated with CQEt (10 g/100 g
diet).
Diet and water were provided ad libitium and body weight was recorded at reg-
ular intervals. At the end of 60 days, the animals were deprived of food overnight,
administrated ketamine hydrochloride (35 mg/kg) and sacrificed by decapitation.
Blood was collected and plasma was separated by centrifugation (1500 g, 15 min,
room temperature). Plasma glucose and insulin levels were determined. Insulin
sensitivity was assayed by calculating homeostatic model assessment (HOMA) val-
ues. Liver was immediately dissected out and washed in ice-cold saline. Liver tissue
homogenate (10%) was prepared using 0.025 M Tris–HCl buffer, pH 7.5 or phos-
phate buffer, pH 7.
2.3. In vivo assays
2.3.1. Glucose, insulin and insulin sensitivity indices
Plasma glucose and insulin were measured using kits obtained from Agappe
Diagnostic Pvt. Ltd., Kerala and Accubind microwells, Monobind Inc., CA, USA,
respectively. IR was assessed by computing HOMA, quantitative insulin sensitivity
check index (QUICKI) and insulin resistance indices FIRI (Shalam et al., 2006). The
formulae used are given below:
HOMA = Insulin (
l
U/mL) glucose (mM)/22.5
QUICKI ¼1
logðglucose mg=dLÞþlogðinsulin mU=LÞ
FIRI ¼ðfasting insulin ð
l
U=mLÞfasting glucoseðmg=dLÞÞ=25:
2.3.2. Marker enzymes
To assess the liver injury, activities of aspartate transaminase (AST), alanine
transaminase (ALT), alkaline phospatase (ALP) and gamma glutamyl transferase
(GGT) were assayed using kits obtained from Agappe Diagnostics, Kerala, India.
2.3.3. Liver lipid levels
Lipids were extracted from liver by the method of Folch et al. (1957). Total lipid,
triglycerides (TG) (Foster and Dunn, 1973), cholesterol (Zlatkis et al., 1953), free
fatty acids (FFA) (Falholt et al., 1973) and phospholipids (Zilversmit and Davis,
1950) were determined.
2.3.4. Lipid and protein damage
Lipid peroxidation was evidenced by measuring the formation of thiobarbitutric
acid reactive substance (TBARS) and lipid hydroperoxide (LHP) in liver samples fol-
lowing the method Niehaus and Samuelson (1968) and Jiang et al. (1992), respec-
tively. The protein carbonyl content in the liver was measured by the method of
Levine et al. (1990).
2.3.5. Antioxidant status
The antioxidant status in plasma and liver was evaluated by estimating the lev-
els of non-enzymatic antioxidants such as reduced glutathione (GSH) by the meth-
od of Ellman (1959), vitamin E was estimated in the lipid extract by the method of
Baker et al. (1980) and vitamin C by that of Roe and Kuether (1942). The activities of
enzymatic antioxidants such as glutathione peroxidase (GPx), superoxide dismu-
tase (SOD) and catalase (CAT) and glutathione S-transferase (GST) were assayed
in both hemolysate and liver by the methods outlined elsewhere (Anitha Nandhini
et al., 2002).
2.3.6. Histopathological analysis
Histology of liver was studied using hematoxylin and eosin (H and E) and oil red
O staining. A portion of the liver was fixed in 10% buffered formalin, dehydrated in
graded (50–100%) alcohol embedded in paraffin. Thin sections (4–5
l
m) were cut
and stained with H and E. For oil red O staining, frozen liver sample was processed
using cryostat and then fixed and stained.
2.4. In vitro assays
2.4.1. Estimation of total phenolic content and vitamins C and E
Total phenolic content in CQEt was determined using Folins–Ciocalteau reagent
by the method of Singleton and Rossi (1965). The values were expressed as mg of
gallic acid equivalents (GAE)/100 g. Potential antioxidant status of the plant extract
was evaluated by estimating the levels vitamin C by that of Roe and Kuether (1942)
and vitamin E by the method of Baker et al. (1980).
2.4.2. Hydroxyl radical scavenging activity
Hydroxyl radical is the most reactive of the free radicals that can cause damage
to proteins, lipids and DNA (Spencer et al., 1994). The hydroxyl radical scavenging
activity of CQEt was determined by the method of Halliwell et al. (1987). The incu-
bation mixture in the total volume of 1 mL contained 0.2 mL of 100 mM potassium
dihydrogen phosphate–potassium hydroxide buffer pH 7.4, varying volumes of
CQEt (10, 20, 30, 40 and 50
l
g/mL), 0.2 mL of 500
l
M ferric chloride, 0.1 mL of
1 mM ascorbic acid, 0.1 mL of 1 mM ethylenediamine tetra acetate (EDTA), 0.1 mL
of 10 mM hydrogen peroxide and 0.2 mL of 2-deoxyribose. The contents were
mixed thoroughly and incubated at room temperature for 60 min. Then 1 mL of
1% thiobarbitutric acid (TBA) (1 g in 100 mL of 0.05 N NaOH) and 1 mL of 28% tri-
chloro acetic acid (TCA) were added. All the tubes were kept in boiling water bathy
for 30 min. Gallic acid and vitamin C were used as a positive control for comparison.
The absorbance was read in a spectrophotometer at 532 nm with reagent blank
2022 J. Chidambaram, A. Carani Venkatraman / Food and Chemical Toxicology 48 (2010) 2021–2029