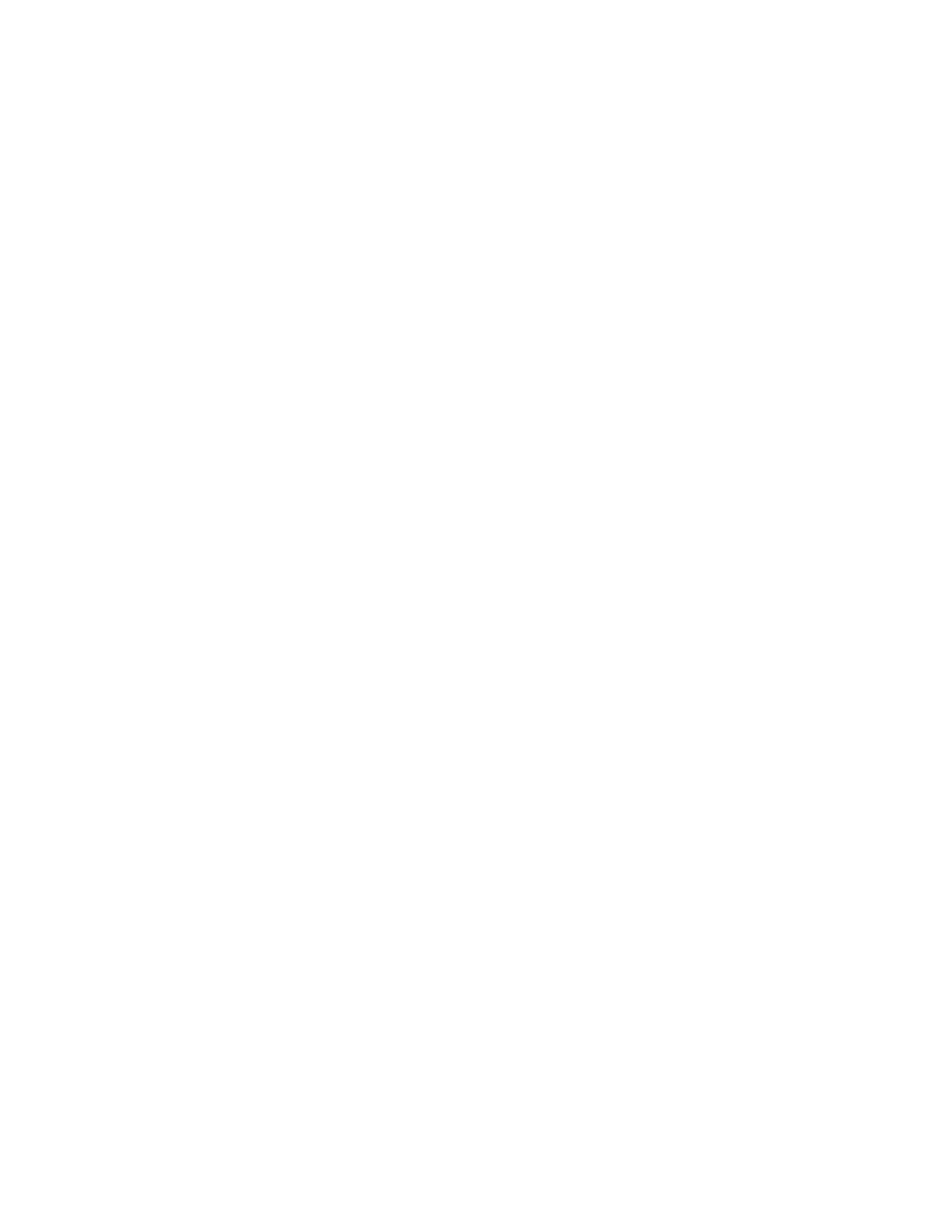
v
v
Abstract
Cardiopulmonary bypass (CPB) triggers a systemic inflammatory response, an
ischemia-reperfusion (I-R) injury and endothelial dysfunction in the pulmonary
circulation. Pulmonary hypertension (PH) is a consequence of this insult. The
latter increases right ventricle work and may cause difficult separation from
cardiopulmonary bypass (CPB) and increased vasoactive requirements after
cardiac surgery. Administration of inhaled milrinone or epoprostenol has been
shown to reduce endothelial dysfunction in the pulmonary artery. The aim of
this work is to evaluate different nebulisators for the administration of
milrinone and to evaluate the effect of pre-emptive treatment with inhaled
milrinone and epoprostenol on postoperative outcome in cardiac surgery.
Two different studies were done. In the first, three groups of swine were
compared: (1) ultrasonic nebulisator inhaled milrinone group; CPB and
reperfusion preceded by 2.5 mg inhaled milrinone, (2) simple jet nebulisator
inhaled milrinone group; CPB and reperfusion preceded by 2.5 mg inhaled
milrinone, and (3) control group; CBP 90 minutes followed by 60 minutes of
reperfusion without treatment. During the procedure, hemodynamic,
biochemical and hematologic parameters were measured. After sacrifice,
pulmonary arterial endothelium-dependent relaxations to acetylcholine and
bradykinin were studied in organ chamber experiments. There was a greater
improvement in endothelium-dependent relaxations to bradykinin and
acetylcholine in the ultrasonic nebuliser inhaled milrinone group compared with
the control group and the simple jet nebulisator inhaled milrinone group.
In the second study, a retrospective analysis of 60 high-risk surgical patients
with PH operated at the Montreal Heart Institute was conducted. Two groups
were compared: (1) 40 patients received both inhaled milrinone and inhaled
epoprostenol before CPB (treatment group); (2) 20 patients with equivalent