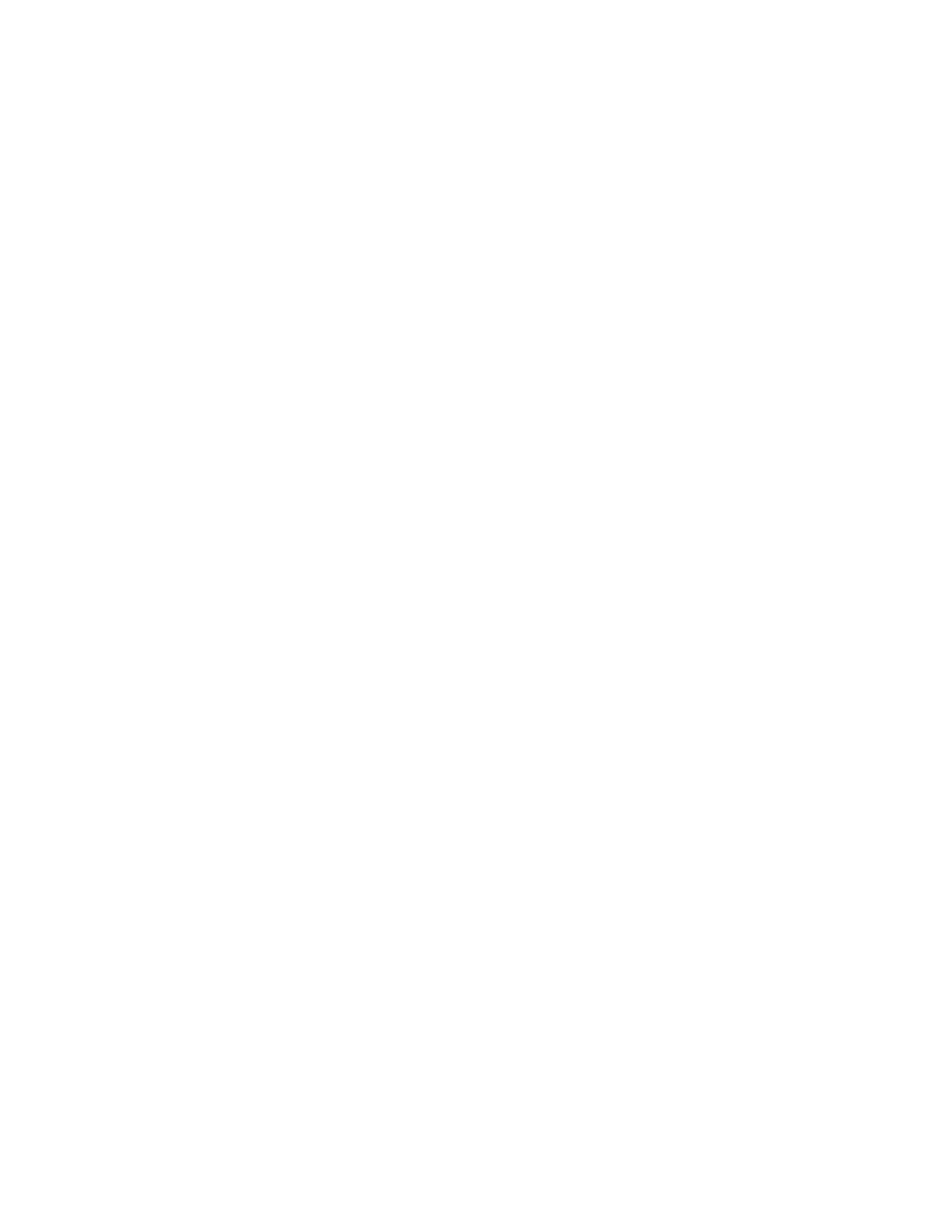
v
Table of Contents
Abstract ............................................................................................................................... ii
Acknowledgements ............................................................................................................ iii
Dedication .......................................................................................................................... iv
List of Figures and Illustrations ....................................................................................... viii
List of Symbols, Abbreviations and Nomenclature .............................................................x
Epigraph ........................................................................................................................... xiii
CHAPTER ONE: INTRODUCTION ................................................................................14
1.1 Breast Cancer: The Clinical Problem ......................................................................15
1.1.1 Breast Cancer Epidemiology ...........................................................................15
1.1.2 Metastatic Disease in Breast Cancer ...............................................................15
1.2 The Metastatic Cascade as it Pertains to Breast Cancer ..........................................16
1.2.1 Prevailing Model of Metastasis .......................................................................17
1.2.2 Seed and the Soil .............................................................................................20
1.2.3 Breast Tumor Microenvironment & Metastasis ..............................................20
1.3 Tumor Endothelial Marker 8 (TEM8) .....................................................................22
1.3.1 Structure ..........................................................................................................22
1.3.2 TEM8 Function ...............................................................................................25
1.4 Preliminary Data ......................................................................................................26
1.4.1 TEM8 Expression in Human Breast Cancer Cell Lines ..................................27
1.4.2 Effect of TEM8 on tumor progression and metastasis in vivo ........................27
1.4.3 Limitation of Observations ..............................................................................27
CHAPTER TWO: HYPOTHESIS AND SPECIFIC AIMS ..............................................29
2.1 Hypothesis ...............................................................................................................30
2.2 Specific Aims ...........................................................................................................30
Aim 1: The prognostic significance of TEM8 in breast cancer will be evaluated. ..30
Aim 2: The in vitro characteristics of breast cancer cells as a function of TEM8
expression will be assessed. .............................................................................30
Aim 3: The in vivo characteristics of breast cancer cells as a function of TEM8
will be assessed. ...............................................................................................31
CHAPTER THREE: MATERIALS & METHODS ..........................................................32
3.1 Cell Lines .................................................................................................................33
............................................................33
3.3 Gateway Lentiviral Constructs ................................................................................34
3.4 Western Blots ...........................................................................................................34
3.5 MTT Cellular Viability Assay on ECM Substrates .................................................35
3.6 Cell Adhesion ..........................................................................................................35
3.7 Cell Migration ..........................................................................................................36
37
3.8 3D Culture Invasion Assays ....................................................................................38
3.9 Apoptosis .................................................................................................................39
3.10 Cell Cycle ..............................................................................................................39