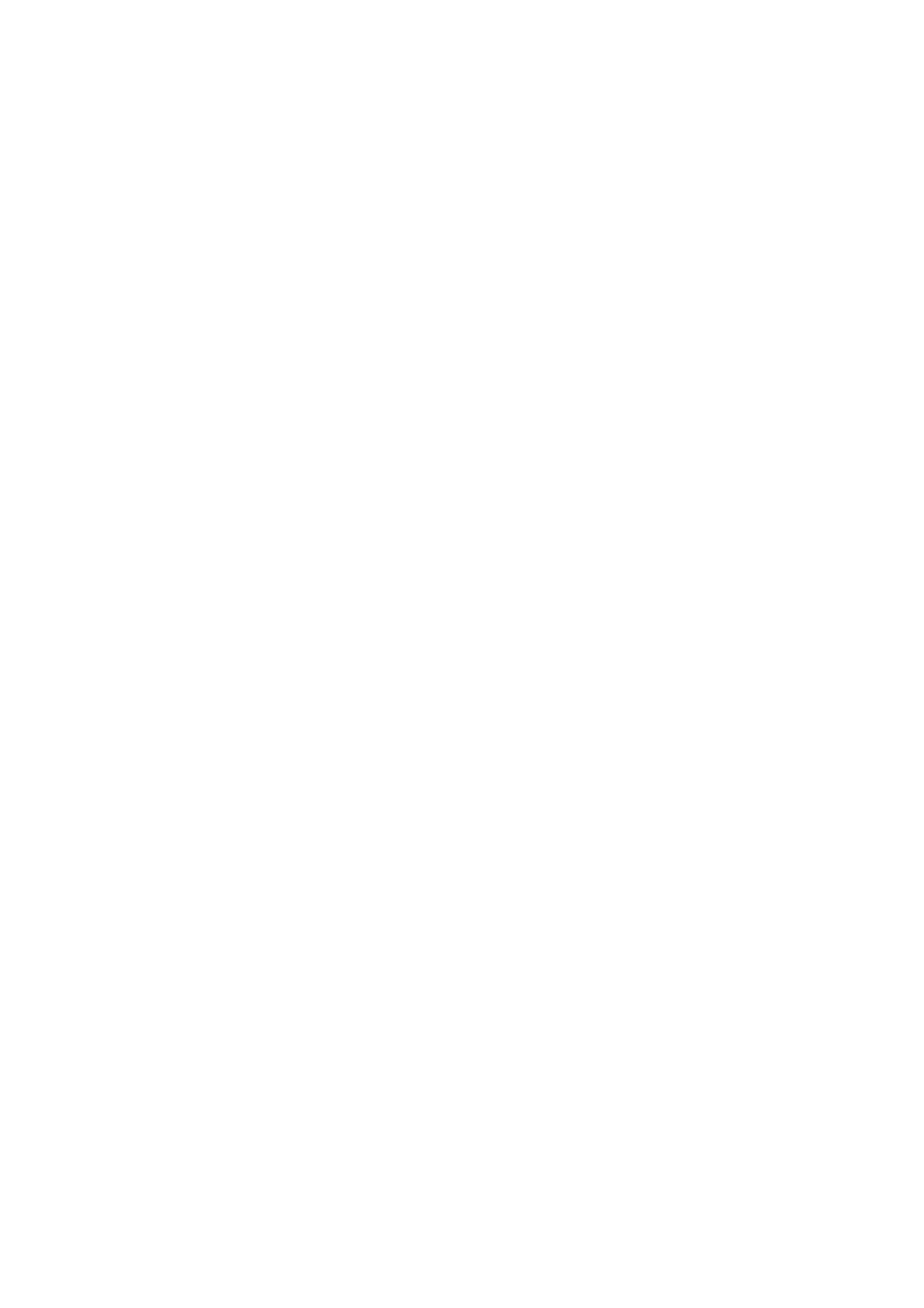
ENRIC MATEU DE ANTONIO i JOAQUIM SEGALÉS I COMA, professors
titulars del Departament de Sanitat i d’Anatomia Animals de la Facultat de
Veterinària de la Universitat Autònoma de Barcelona.
Certifiquen:
Que la memòria titulada, “Characterization of immune responses to porcine
circovirus type 2 (PCV2) infection and vaccination in pigs” presentada per
Maria Fort de Puig per l’obtenció del grau de Doctor en Veterinària, s’ha
realitzat sota la seva direcció a la Universtitat Autònoma de Barcelona.
I per tal que consti als efectes oportuns, signen el present certificat a Bellaterra, a
15 de juny de 2009.
Dr. Enric Mateu de Antonio Dr. Joaquim Segalés i Coma