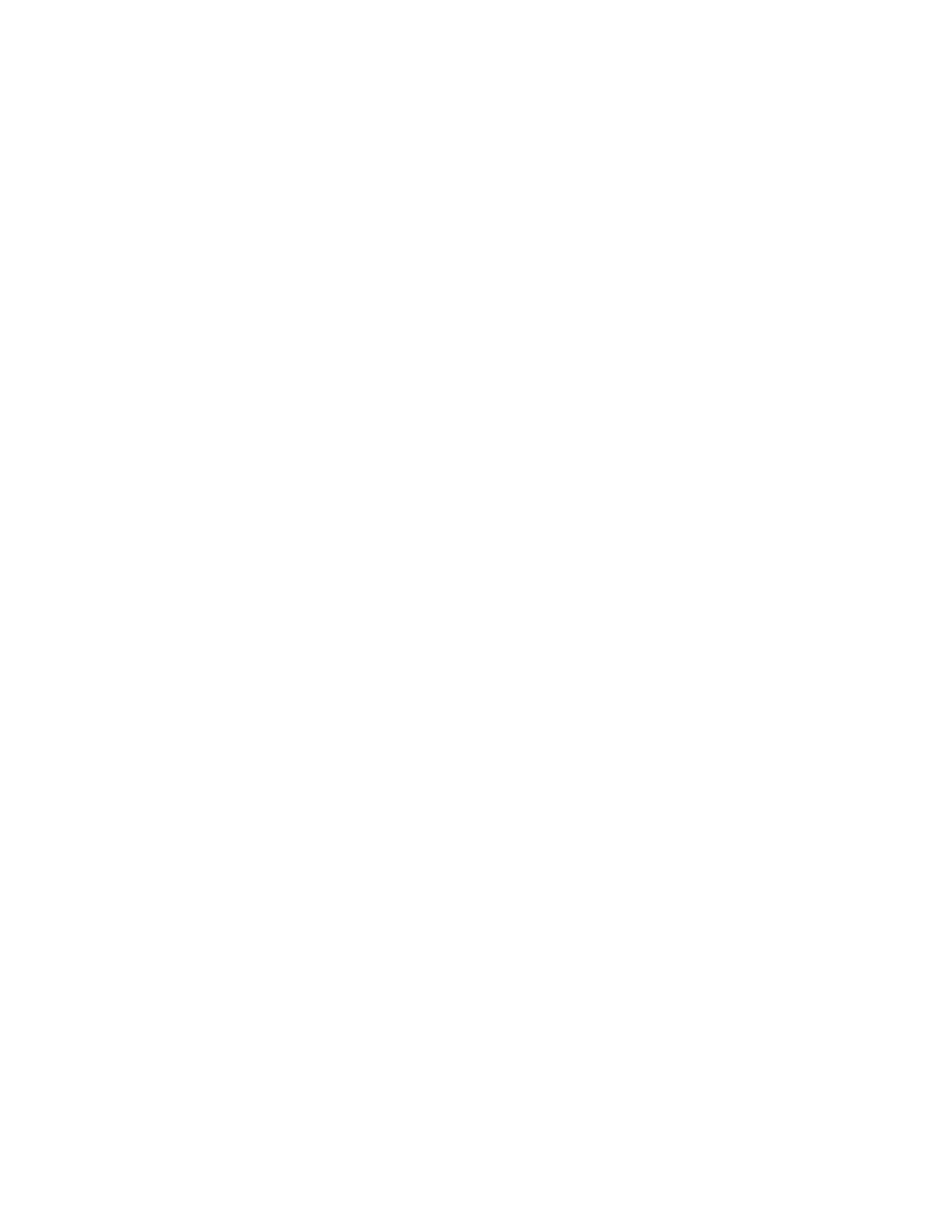
iii
Joaquim Segalés i Coma, professor titular del Departament de Sanitat i
d’Anatomia Animals de la Facultat de Veterinària de la Universitat Autònoma
de Barcelona i director del Centre de Recerca en Sanitat Animal (CReSA) i
Marina Sibila i Vidal, investigadora del Centre de Recerca en Sanitat Animal
(CReSA),
CERTIFIQUEN:
Que la Tesi doctoral titulada “New insights on PCV2 vaccination: thinking out
of the box” presentada por Hua Feng per a l’obtenció del grau de Doctor en
Veterinària, s’ha realitzat sota la nostra supervisió al Centre de Recerca en
Sanitat Animal.
Per tal de que consti, als efectes oportuns, signen el present certificat a
Bellaterra (Barcelona), a 17 de Setembre de 2015.
Dr. Joaquim Segalés i Coma Dr. Marina Sibila i Vidal
Hua Feng