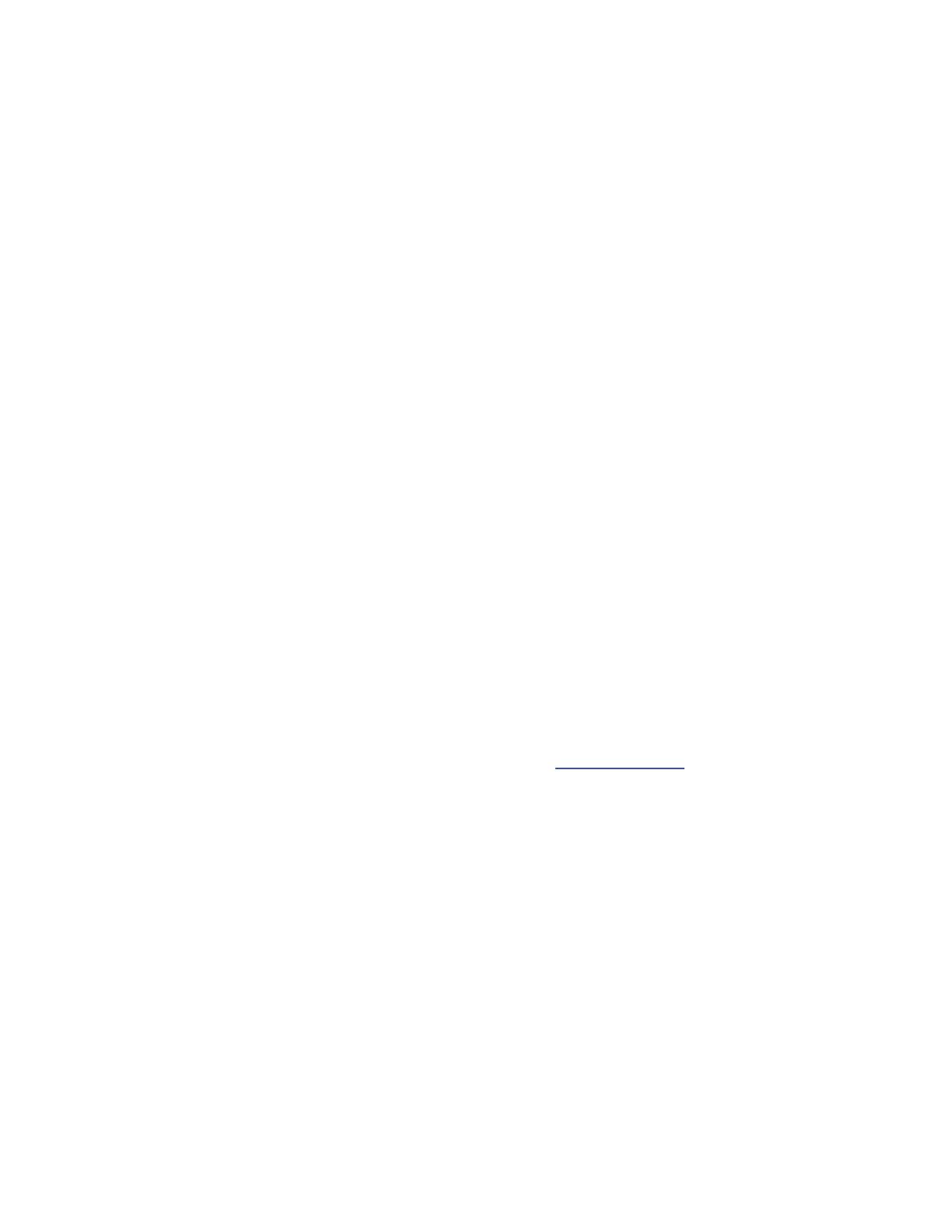
1
Phosphorylation at Ser-181 of oncogenic KRAS is required for tumor growth 1
Carles Barcelóa*, Noelia Pacoa*, Mireia Morellb, Blanca Alvarez-Moyaa,d, Neus Bota-2
Rabassedasa,e, Montserrat Jaumota, Felip Vilardellc, Gabriel Capellab and Neus Agella,1 3
aDepartament de Biologia Cel•lular, Immunologia i Neurociències, Institut 4
d’Investigacions Biomèdiques August Pi i Sunyer (IDIBAPS), Facultat de Medicina. 5
Universitat de Barcelona, C/ Casanova 143, 08036 Barcelona, Spain 6
bHereditary Cancer Program, Translational Research Laboratory, Catalan Institute of 7
Oncology, ICO-IDIBELL, Hospitalet de Llobregat, Barcelona, Spain; Gran Via 199-8
203, 08907- L’Hospitalet de Llobregat Spain 9
c Servei d’Anatomia Patològica i Institut de Recerca Biomèdica de Lleida, Avda. Rovira 10
Roure, 80, 25198 Lleida. 11
d Present address: IFOM-IEO Campus, Milan, Italy 12
e Present address: IBE, CSIC-UPF, Barcelona, Spain 13
*Both authors contributed equally to this work 14
1Corresponding author: Neus Agell, Dept. Biologia Cel·lular, Immunologia i 15
Neurociències, Facultat de Medicina, Universitat de Barcelona, C/Casanova 143, 16
Running title: KRAS phosphorylation promotes tumor growth 18
Keywords: KRAS, tumorogenesis, phosphorylation, PKC 19
Grant support: This study was supported by MICINN-Spain [SAF2010-20712] 20
Conflict of interest: none 21
Word count : 5150 22
Number of figures: 7 23
24
Research.
on January 7, 2014. © 2013 American Association for Cancercancerres.aacrjournals.org Downloaded from
Author manuscripts have been peer reviewed and accepted for publication but have not yet been edited.
Author Manuscript Published OnlineFirst on December 26, 2013; DOI: 10.1158/0008-5472.CAN-13-1750